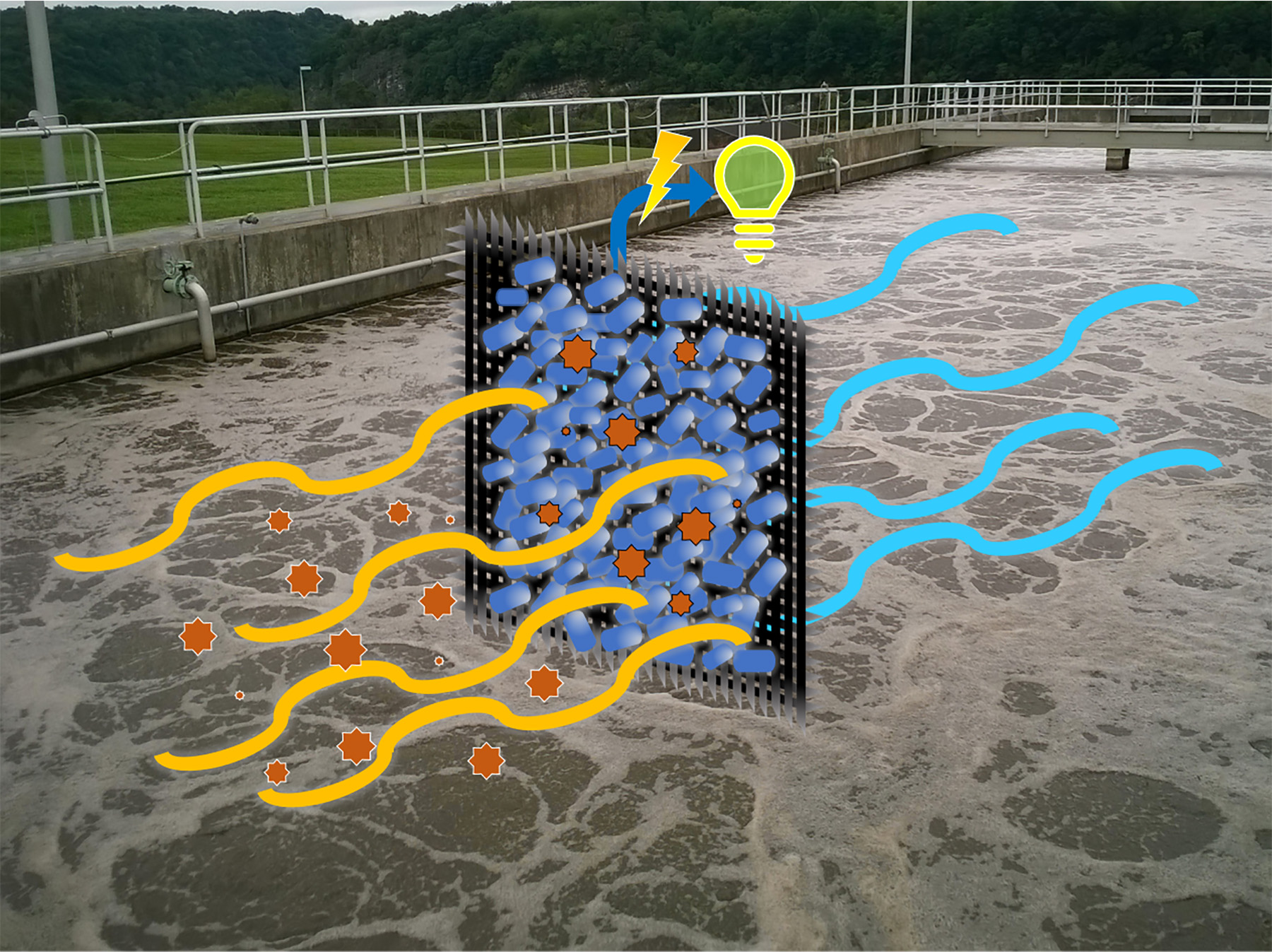
By Robert L. Reid
Researchers have long known how to use the bacteria in wastewater to generate energy — whether biogas or bioelectricity — and to recover the nutrients from the organic material, says Zhen “Jason” He, Ph.D., a professor in the Department of Energy, Environmental & Chemical Engineering in the McKelvey School of Engineering at Washington University in St. Louis. But these methods usually did so “at the expense of the water,” which could have been filtered and reused but was not, He explains.
This was because there has often been little incentive to recover the water from wastewater, especially not in developed countries where water can be supplied relatively inexpensively. More recently, however, concerns about climate change and the push for greater sustainability are promoting the recovery of as many resources as possible from waste materials. “And the biggest resource from wastewater is, of course, the water,” He explains.
To that end, He and his Washington University colleagues have developed a bench-scale wastewater treatment system that features a microbial fuel cell with a dual-function electrode that combines the processes of energy production and water filtration. The primary goal of these developments is enhanced wastewater treatment, so the generation of electricity and the production of reusable water are considered bonuses.
Forming a fuel cell
According to information from the U.S. Department of Energy, a fuel cell “consists of two electrodes — a negative electrode (or anode) and a positive electrode (or cathode) — sandwiched around an electrolyte. A fuel, such as hydrogen, is fed to the anode, and air is fed to the cathode.” In a traditional fuel cell, a noble metal such as platinum serves as the catalyst material to produce electricity from the hydrogen and oxygen. For a microbial fuel cell, electrochemically active bacteria act as the catalyst. What makes the Washington University system unique is that a permeable anode electrode serves as both an electrode and as the filtration media, He says.
The permeable anode features a dynamic membrane made from a conductive carbon cloth composite material sewn together with a stainless steel mesh using titanium wire. The carbon cloth membrane allows bacteria to grow on its surface, forming a biofilm, while also enabling the wastewater to flow from the area known as the anaerobic chamber to the anode chamber, He says.
The stainless steel mesh system functions as a current collector and supporter for the anode electrode. Working together, the bacteria and the membrane can remove as much as 90% of the organic materials. This process generates water that is clean enough to either be discharged or further treated for nonpotable uses such as flushing toilets or irrigation.
It is possible to treat the water to a potable standard, He adds, but the process is quite expensive and much of the public is not prepared to drink former wastewater, “no matter how clean it is.”
Testing times three
In the Washington University experiments, He and his colleagues constructed three bench-scale reactors — capable of accommodating just a few hundred milliliters of wastewater — labeled A, B, and C. The A reactor featured the carbon cloth and stainless steel mesh membrane, and the wastewater flowed from the anaerobic chamber through the composite filtering membrane into the anode chamber. In the B reactor, the influent went straight from the anaerobic chamber into the anode chamber without passing through the filter membrane to test the function of the filtration media. The B reactor membrane thus acted only as the anode electrode. In the C reactor, the carbon cloth membrane did not feature the stainless steel mesh and thus did not serve as an anode electrode.
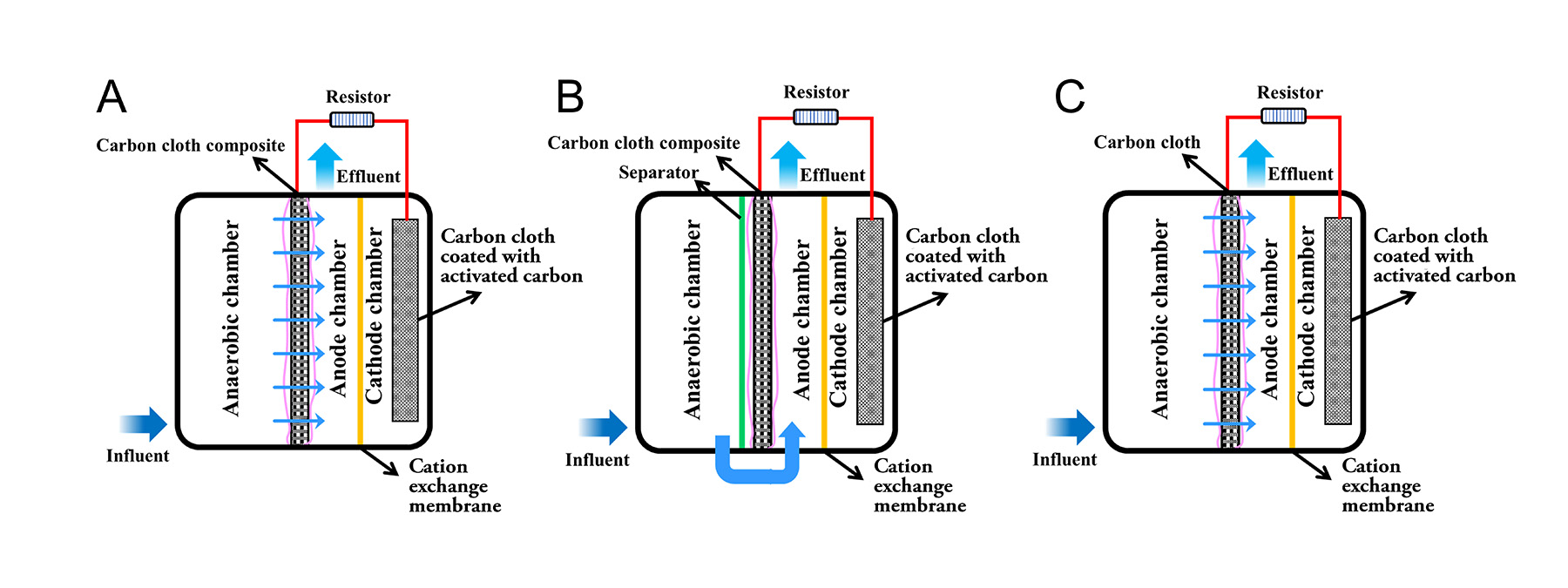
Each experiment was conducted multiple times, using an anaerobic sludge from two wastewater treatment plants, one in Blacksburg, Virginia, and the other in St. Louis. Initially, the sludge was cultivated with a synthetic wastewater to “start the reactors” because of the known qualities of the synthetic material. “You know what’s inside, you know there are enough nutrients,” He explains.
Those known qualities “let us verify that the reactors work well ... and the design is OK,” He says. In real wastewater, “you’re not certain. It really depends on what we dump into the sewer system.”
Once the design of the experiments had been verified, later tests were conducted using actual domestic wastewater, including one test that lasted 30 days.
Verifying performance and looking ahead
During the experiments, the researchers monitored the voltage generated, measured the turbidity of the water, analyzed the removal of organic contaminants, and tested the effects of using multiple layers of dynamic membranes, among other actions. The findings appeared in the article “Enhancing the Performance of a Microbial Electrochemical System with Carbon-Based Dynamic Membrane as Both Anode Electrode and Filtration Media,” which was the cover story in the May 2021 issue of the scientific journal Environmental Science: Water Research & Technology, published by the Royal Society of Chemistry in Cambridge, England, United Kingdom.
During the experiments, the researchers monitored the voltage generated, measured the turbidity of the water, analyzed the removal of organic contaminants, and tested the effects of using multiple layers of dynamic membranes, among other actions. The findings appeared in the article “Enhancing the Performance of a Microbial Electrochemical System with Carbon-Based Dynamic Membrane as Both Anode Electrode and Filtration Media,” which was the cover story in the May 2021 issue of the scientific journal Environmental Science: Water Research & Technology, published by the Royal Society of Chemistry in Cambridge, England, United Kingdom.
The results helped verify the enhanced wastewater treatment performance of the dynamic membrane as a filtering material and the use of the stainless steel mesh to make the anode work as an electrode. Although the estimated energy production was not high — the system can generate 0.02 kWh when treating 1 cu m of wastewater — the system itself “would consume much less energy than traditional treatment systems,” He explains. The energy produced “may be used to further offset energy consumption” in the wastewater treatment process.
Looking ahead, He would like to scale up his experiments and conduct longer-term operations of the system. The experiments so far also identified various aspects of the project in need of additional research, including how to prevent fouling, which are blockages of the filtering membrane; how to handle nutrients and microbes; and other methods of improving the performance of the system.
Robert L. Reid is the senior editor and features manager of Civil Engineering.
This article first appeared in the January/February 2022 issue of Civil Engineering.