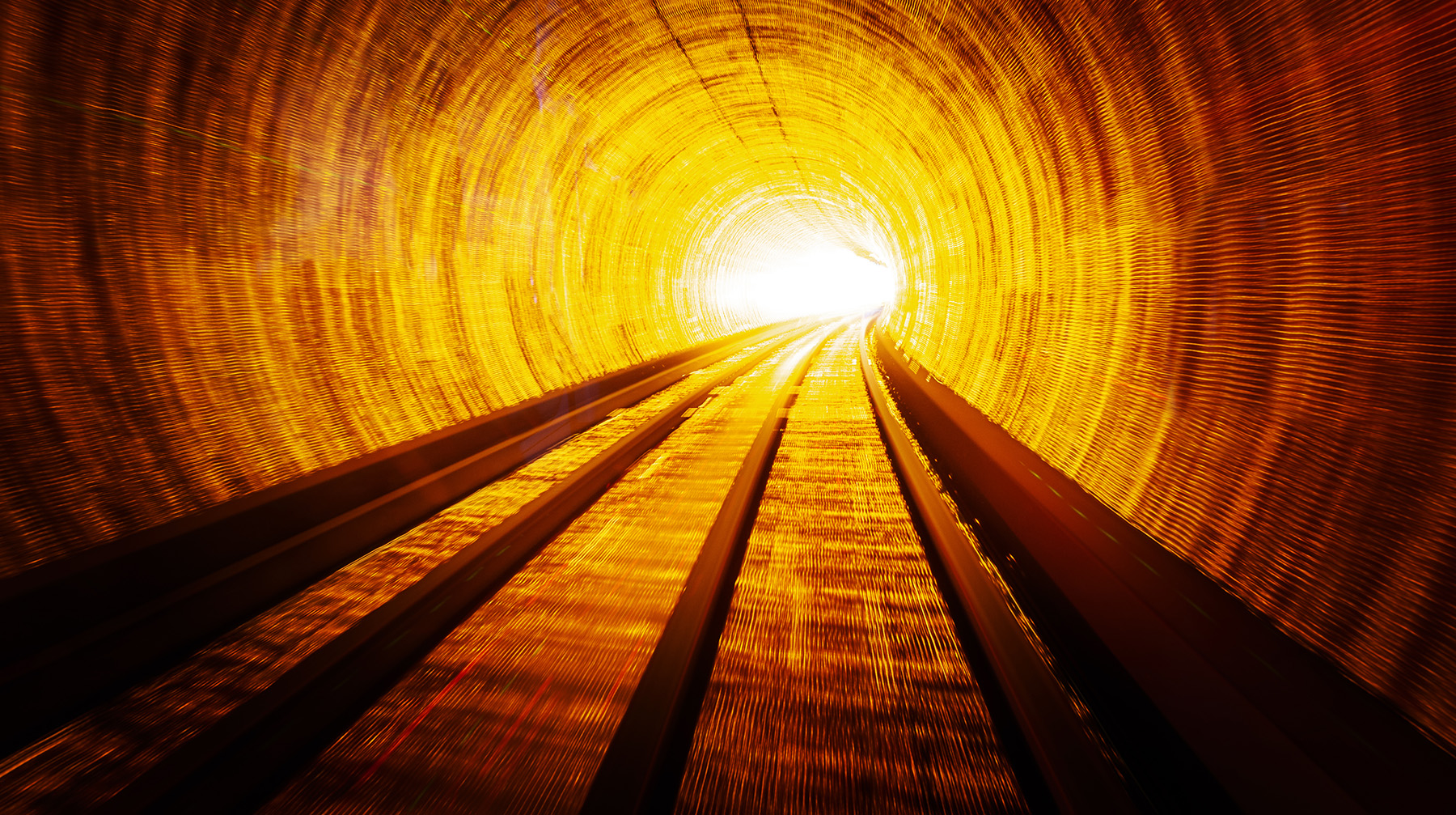
By Ian Chaney, P.E.
Designing underground structures, especially road and rail networks, that are resilient to seismic events is a complex process. WSP engineers who have designed and constructed such infrastructure offer insight into these types of projects.
As cities and urban areas grow, so does the need to expand road and rail networks to move people and goods efficiently. Because the surface space is already in use, much of this new transportation infrastructure must be constructed underground. These belowground projects alter communities in positive ways, connecting neighborhoods and providing a cohesiveness that binds these communities together.
Designing and building underground infrastructure, such as tunnels and shafts, requires unique perspectives, experience, and equipment. Design, engineering, and construction teams must work in constrained and challenging settings: avoiding or rerouting utilities, navigating around building foundations, and assessing and managing impacts on and from adjacent infrastructure — in addition to the sophisticated geotechnical analyses required to build major underground projects in such critical areas.
This article provides insights from WSP engineers who have designed and delivered major rail tunnel projects in seismically active areas of the United States.
Designing for risk tolerance
Geotechnical engineers, seismologists, geologists, and other experts often work with transit and transportation agencies in seismically active regions to help them develop a design approach that will achieve seismic resilience for the underground and aboveground infrastructure they wish to build.
Generally, these approaches involve consideration of two levels of earthquake intensity, which are based on probabilistic seismic hazard assessments performed for each project site. The lower-level intensity prepares for relatively common and moderate seismic events: underground transit or road networks are expected to experience little to no damage and will resume operations after pausing briefly for damage inspections.
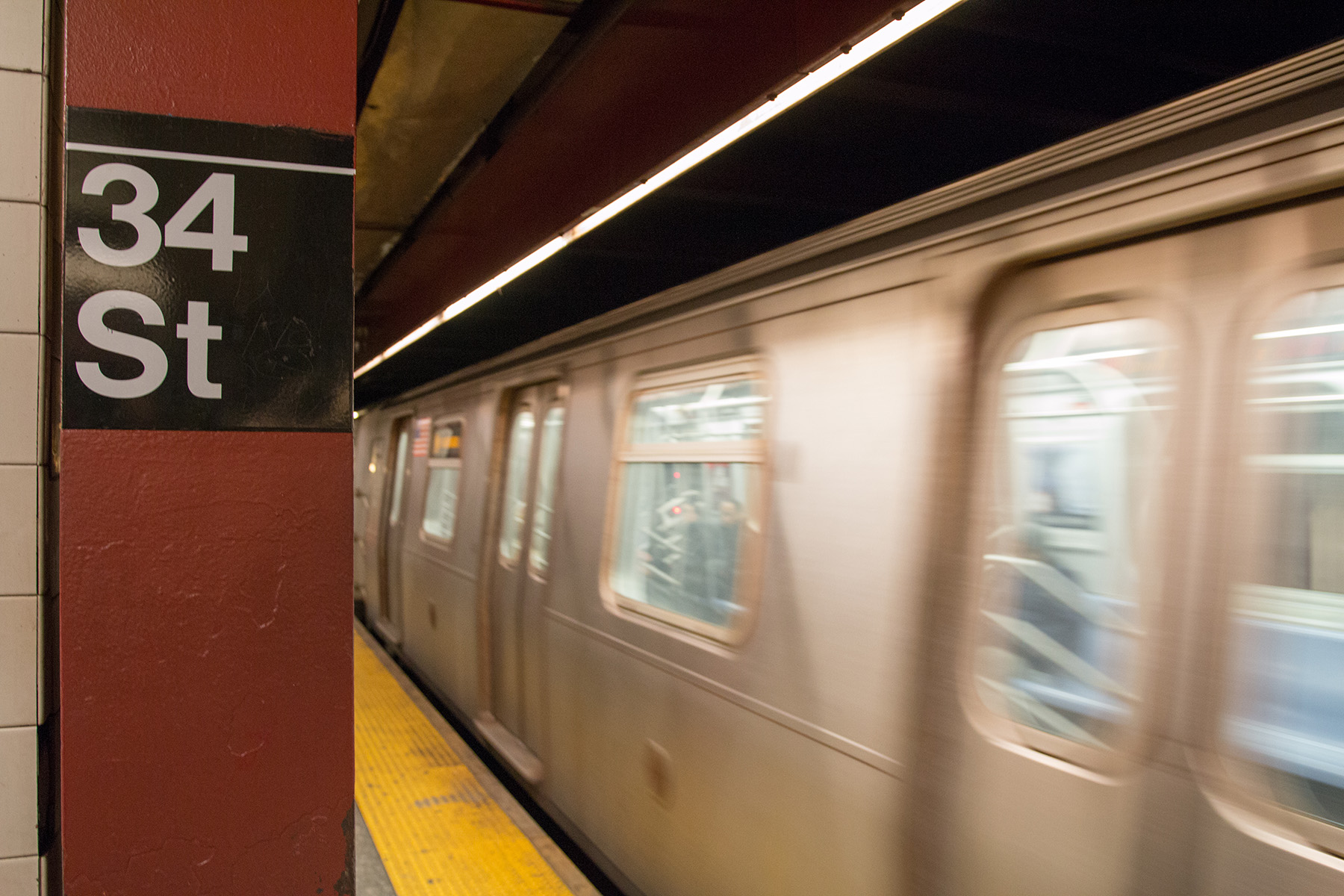
Higher-intensity earthquakes are more severe but less frequent. The performance criteria for this type of event consider the safety of occupants, collapse prevention, and renewal of service within a reasonable amount of time —factors that take into account an owner’s return-to-service requirements.
For example, an owner may define a lower-level earthquake as an Operating Design Earthquake with a 50% probability of exceedance in 100 years (a return period of 150 years) and a higher-level Maximum Considered Event Earthquake as an event with a 4% probability of exceedance in 100 years (a return period of 2,500 years). These and other seismic performance requirements often stipulate a time frame in which damage must be repaired, as well as goals for resuming operations.
Numerical modeling is used to estimate the possibility and severity of ground deformations due to ground shaking, liquefaction, surface faulting, and slope failure. These analyses can be used to develop mitigation measures. For liquefaction, mitigation techniques may include ground improvement, such as grouting, stone columns, or densification. For surface faulting, measures may include special structural detailing of the tunnel lining. And for slope stabilization, common mitigation techniques encompass soil reinforcement and retaining walls, among other measures.
“An important consideration for a seismically resilient tunnel is adequate ductility to ensure the lining can deform without significant damage,” says Steve Klein, P.E., G.E., M.ASCE, senior engineering manager at WSP. “This is the key to meeting performance objectives and returning the tunnel to service as soon as possible after a major seismic event. Finding the right balance between the allowable amount of inelastic behavior and the time to repair the tunnel is fundamental to achieving a resilient tunnel structure.”
Urban complexities ...
The San Francisco Municipal Transportation Agency’s newest subway line is the Central Subway (shown at the bottom of the figure), which extends its Muni Metro T Third line 1.7 mi, adding four new stations that connect some of the city’s busiest districts. Planning began in 2009, and construction occurred from 2011 to 2021. Revenue service for this line began in January 2023. To build the line, WSP-led design teams used pressurized-face tunnel boring machines to construct twin tunnels through saturated sands along a curved alignment only 12 ft below the active Bay Area Rapid Transit tunnels.
A major consideration of this project was how to minimize settlement of the BART tunnels and prevent impacts to the existing Muni subway line tunnels during construction. WSP used the TBMs in conjunction with compensation grouting, a technique that injects cement grout under pressure to control ground settlements caused by tunneling. The team monitored the BART tunnels continuously and met daily with the contractor, construction manager, program manager, and BART staff while the two TBMs passed below.
Some areas in the new tunnels were as deep as 120 ft, which added further complications to the project. In underground terrain projects in which there are existing subway lines and deep foundations, corridor alignments can also require specialized TBMs — as in the case of the Central Subway — that can excavate tighter-radius curves and steeper grades.
Los Angeles County Metropolitan Transit Authority’s Regional Connector Transit Project in downtown Los Angeles, which began service in June 2023, faced similar challenges. WSP provided on-site design support to the project. To build the new 1.9 mi light rail link, a construction joint venture between the tunnel contractors specified a specialized TBM that could accommodate tight curves (minimum radius of 450 ft) in the alignment.
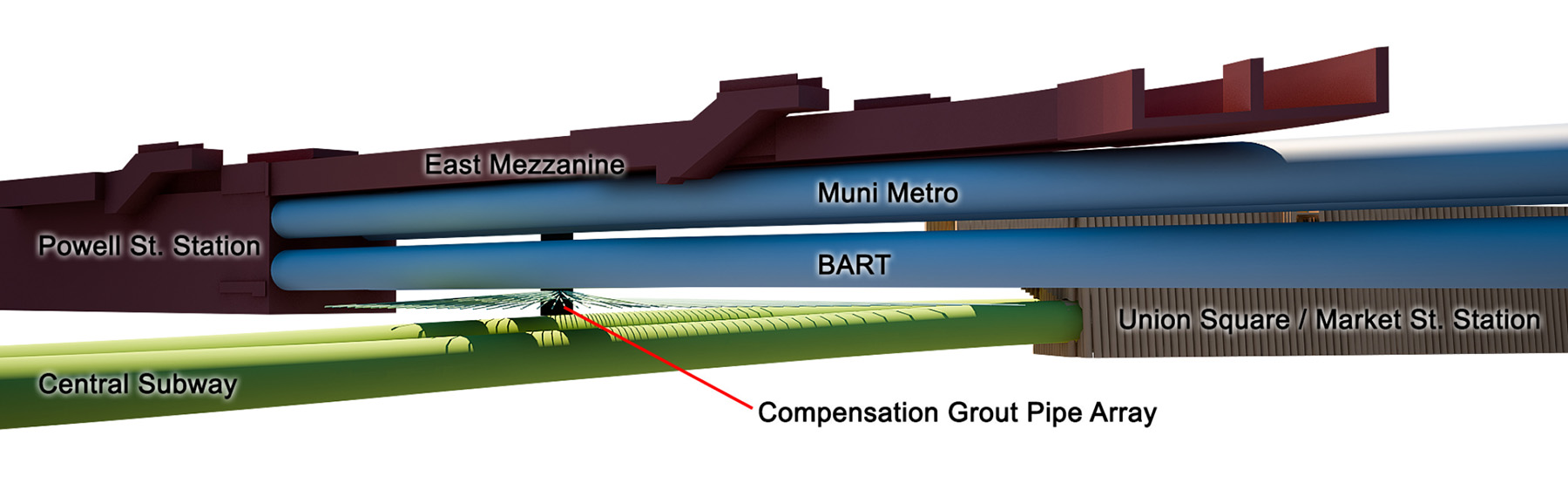
The tunnel launch passed through alluvium soils in a downward-sloping curve with less than 15 ft of cover from the tunnel crown to the building footings above. To mitigate the potential for building settlement, the team employed permeation and compensation grouting.
Instrumentation measurements and TBM performance data were integrated into dashboards, which facilitated the project team’s management of the tunneling operation. For example, a horizontal inclinometer provided unique measurements of ground movement above the tunnels, helping the team deliver a successful launch.
... Meet seismic realities
Designing for earthquake resilience in seismically active regions like western North America has been a central focus of many in the engineering profession for decades. While earthquakes in the Midwest and East are much less common and damaging than in the West, the 4.8 magnitude 2024 Tewksbury, New Jersey, earthquake in April, felt up the East Coast from Virginia to Massachusetts, reminded engineers and infrastructure owners across the country that seismic modeling must always be part of tunnel design, no matter where it is planned.
Although some may find it surprising, underground rail and road tunnels perform well in seismic events. “There’s a fundamental difference between how underground and aboveground structures respond to earthquakes,” says Matt Fowler, P.E., M.ASCE. Fowler is senior vice president of WSP’s tunnels and underground structures division.
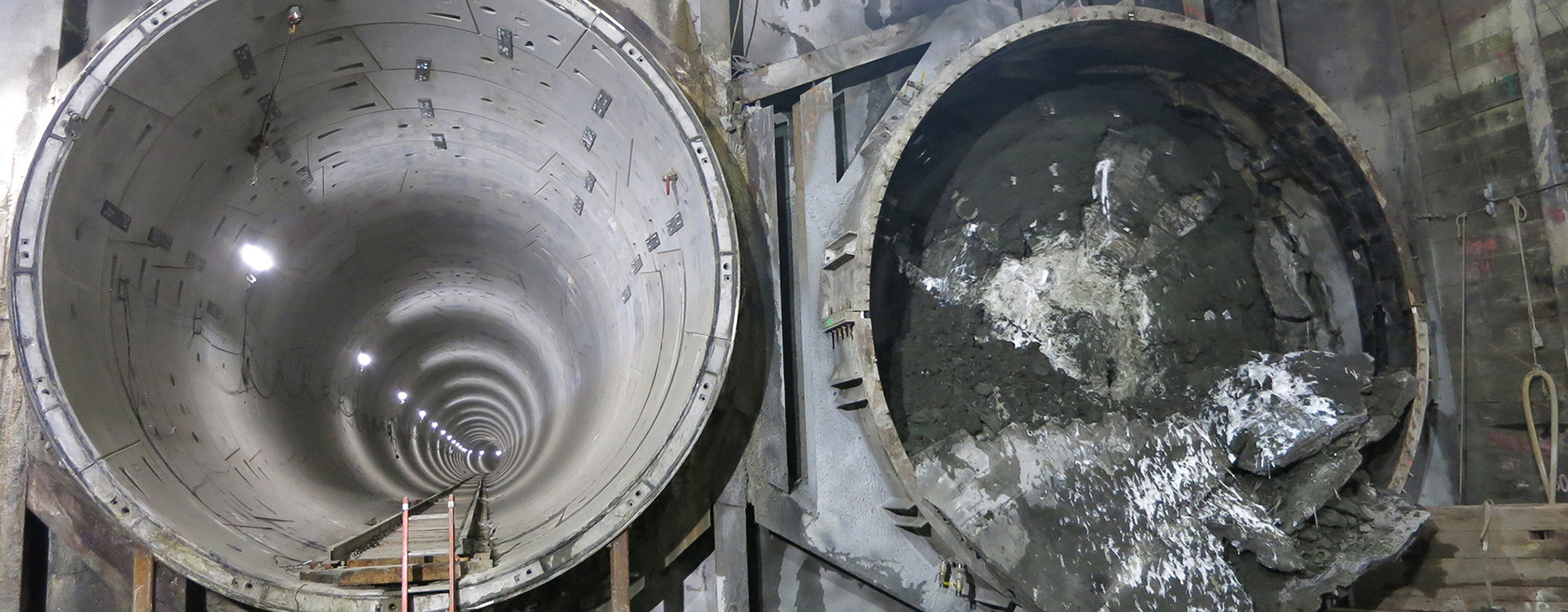
“When the ground is shaking, aboveground structures are subject to inertial forces — the top of a skyscraper, for example, might move by several inches or even feet,” Fowler explains. “Underground structures interact and deform with the seismic waves passing through the ground, and a passenger on a subway may not even feel an earthquake that causes damage aboveground.”
Major earthquakes over the last several decades — such as the 6.9 magnitude 1989 Loma Prieta earthquake in California, the 6.9 magnitude 1995 Kobe earthquake in Japan, and the 7.7 magnitude 1999 Chi-Chi earthquake in Taiwan — have provided robust opportunities to study the responses to and impacts of earthquakes on tunnels and underground stations that have been designed and built to higher seismic standards.
Soil type
Much of this research has focused on soil-structure interaction. Soil characteristics factor significantly into how tunnels will perform in earthquakes. While geologic conditions and soil properties in urban environments are often well understood, seismic response can only be accurately assessed by performing detailed soil-structure interaction modeling.
Creating site-specific representations of ground conditions is critical. Geotechnical or structural engineers typically apply computer models to simulate the ground motions they expect would be associated with earthquakes of varying intensity. Analyses of the models reveal how a structure might respond to ground shaking, including the axial forces and flexural stresses induced in a structure by the seismic waves traveling through the ground.
In some cases, tunnel corridors can be routed through more favorable geology, such as competent rock and stable soil deposits. Tunnels for the City Rail Link in Auckland, New Zealand, for example, were aligned to traverse the East Coast Bays Formation, a geologic group composed of sandstone and mudstone, which are low-strength but largely competent rock.
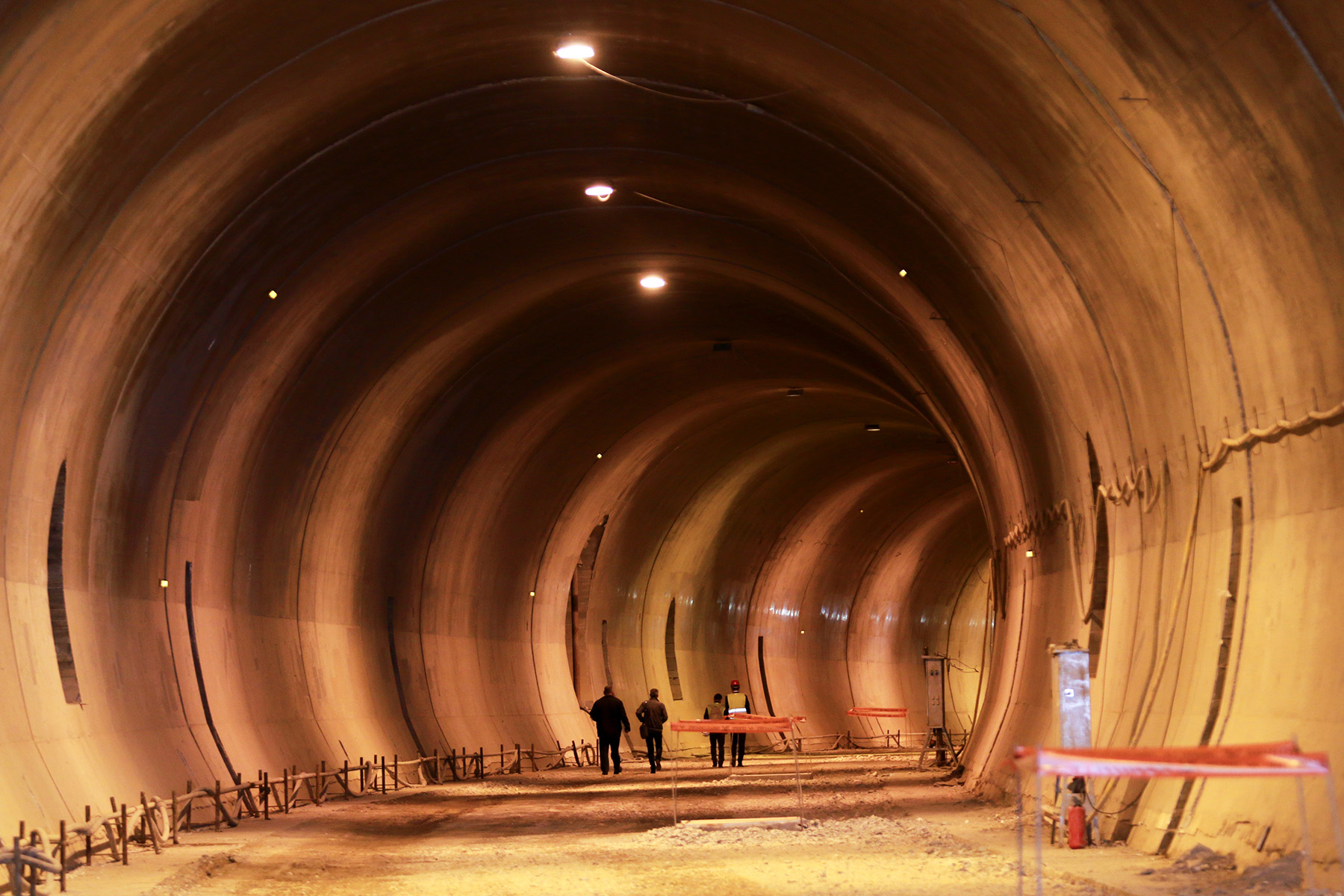
The WSP project team conducted sensitivity analyses to account for ground variability and unexpected conditions and evaluated tunnel performance for a seismic event that exceeded the level required by New Zealand’s design code.
Crossing faults
Building rail and road tunnels across fault lines is obviously not preferable for design and engineering teams, but sometimes it is necessary.
In these cases, design strategies include using special structural detailing, such as shorter precast lining segments, customized connectors, higher-range gaskets, and hybrid reinforcement in the lining. This detailing enhances structural performance to accommodate ground movement, mitigating the chance of tunnel collapse, protecting the lives of those traversing the affected sections, and enabling damage to be repaired and service restored as quickly as possible.
Another common measure is to enlarge the tunnel to provide additional space to repair it and realign the tracks or traffic lanes following a rupture of a fault.
Seismic isolation devices are another option for building underground transit stations. Rubber bearings, for example, absorb seismic energy and allow movement between a tunnel’s internal structures and the lining that deforms with the ground. Friction bearings use sliding surfaces to dissipate seismic energy, and spring systems absorb shocks.
Such methods have been used on a variety of critical infrastructure facilities in seismically active regions, like Los Angeles and the San Francisco Bay Area, and they are approaches that designers of underground transit and rail tunnels may consider when a subterranean rail line is on or near an active fault.
Maximizing resilience
To achieve seismic resilience on the LA Regional Connector Line, the design team engineered the tunnels by evaluating modeled distortions against concrete performance so that the tunnels could withstand the ovaling and axial-curvature responses that could occur during an earthquake.
In the 2022 white paper “Seismic Design Approach for Underground Structures of Los Angeles Metro Regional Connector Transit Corridor Project,” WSP’s Jason Choi and William Hansmire, Ph.D., P.E., F.ASCE, along with Zsolt Horvath, P.E., and Carlos Herranz, P.E., CEng, from Mott MacDonald, stated that the design team used double rubber gaskets (one near the intrados, or interior curve of an arch, and another near the extrados, or exterior curve of an arch) on all segment-mating faces.
High-strength bolts secured the radial joints, and circumferential joints were equipped with special dowels to connect adjacent segment rings. Dowels are a key element within LA Metro tunnel linings, so the team performed rigorous checks on dowel tensile resistance and on concrete breakout capacity associated with the dowels’ pullout force in an earthquake.
The crossover cavern was the most technically complex part of the Regional Connector project. The seismic design of the cavern’s final lining was based on a dynamic time history analysis performed in a 2D, plain-strain soil-structure interaction model using FLAC2D software. The design team developed three horizontal time histories based on the 7.2 magnitude 1992 Cape Mendocino (California) earthquake and the Kobe and Loma Prieta earthquakes.
As required by LA Metro Rail design criteria, the seismic demands due to vertical ground motions were applied using a pseudostatic approach consistent with the relationship between horizontal and vertical accelerations recorded in the vicinity of the Metro tunnels during the 1994 Northridge earthquake.
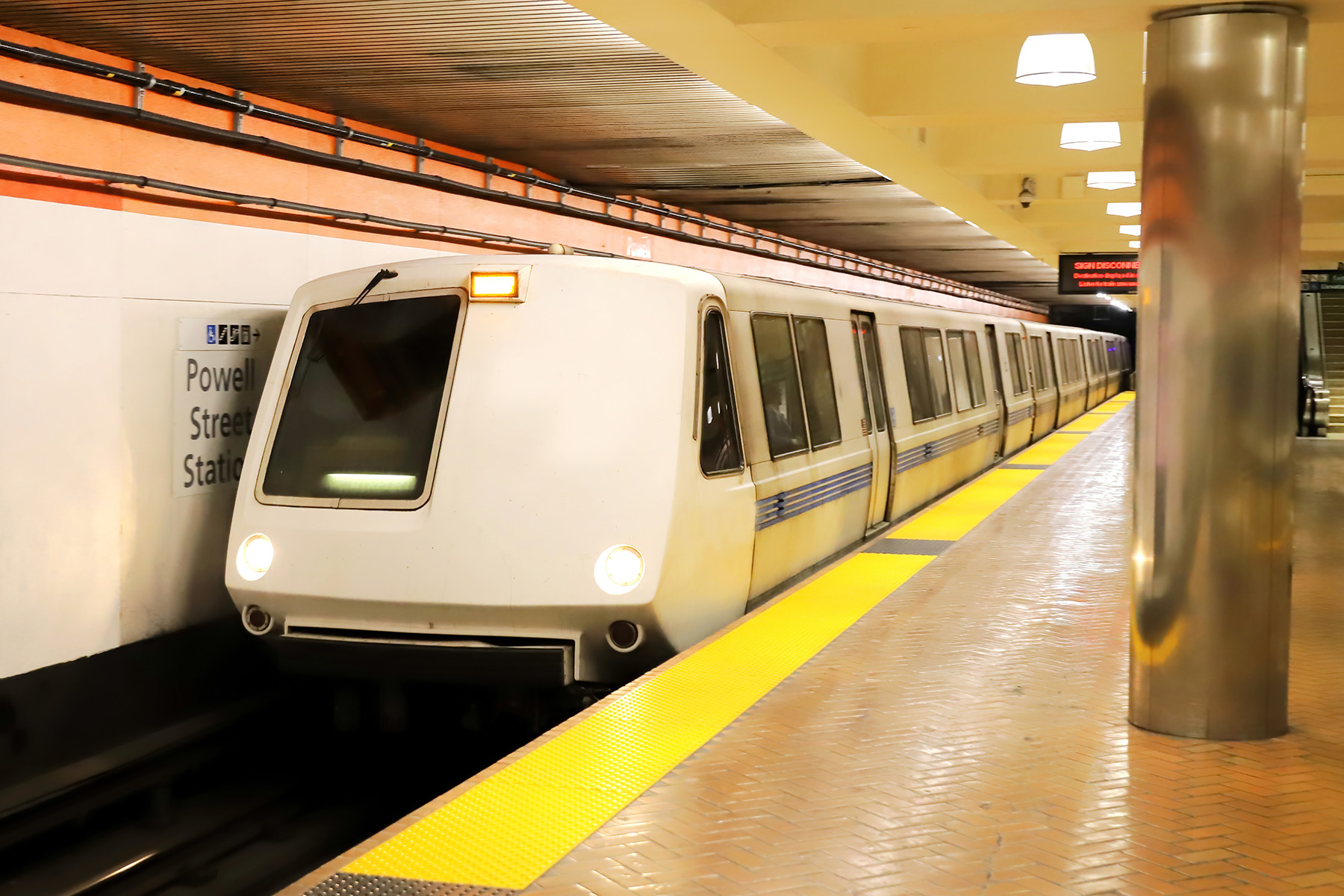
“The final lining in the (numerical) model was simulated with elastoplastic structural elements. During the dynamic analysis, if at any node the thrust-moment combination reached the design capacity of the section, a hinge was automatically introduced at the node and the calculation was continued, leading to a redistribution of stresses to the adjacent sections,” explained the paper’s authors.
“At the locations where plastic hinges were formed, the corresponding section curvature was checked against the allowable limit. The model also included the interior structures consisting of center divider wall and a ventilation plenum slab supported on corbels near the crown.”
A legacy of fundamentals
The BART project for the San Francisco Bay area, designed during the 1960s and opened to service in 1972, was a particularly significant and formative period for the advancement of design methods for tunnels and underground structures in high-seismic areas. The lessons and basic understanding developed for BART continue to be refined and advanced for the LA Metro region and other projects in high-seismic zones throughout the world.
One milestone in research that is still a go-to reference today is Seismic Design of Tunnels: A Simple State-of-the-Art Design Approach, the 1993 seismic design monograph by Jaw-Nan (Joe) Wang, Ph.D., P.E., then an associate of Parsons Brinckerhoff Quade and Douglas Inc. Wang researched how earthquakes affect underground structures, compared seismic design philosophies, and developed simplified methods for evaluating the ovaling and racking effects of earthquakes on tunnels and underground structures.
“Wang’s research and the resulting paper have been put to use all over the world, from Taiwan to California,” says Fowler. “It’s a foundational document that can serve both as a primer for engineers who are new to the field and as a resource for veterans of seismic resilience design for tunnels.”
Tunneling in urban areas at risk of seismic activity adds layers of complexity to already difficult engineering projects. With the foundational work of the past and the careful, innovative, and proven techniques of today, project owners can move forward with unshakable confidence.
Additional reading
Seismic Design of Tunnels: A Simple State-of-the-Art Design Approach by Jaw-Nan (Joe) Wang, Ph.D., P.E.
“Seismic Design Approach for Underground Structures of Los Angeles Metro Regional Connector Transit Corridor Project” by Jason Choi, William Hansmire, Ph.D., P.E., F.ASCE, Zsolt Horvath, P.E., and Carlos Herranz, P.E., CEng
Ian Chaney, P.E., is the geotechnical and tunneling market leader at WSP and is based out of the firm's Virginia Beach, Virginia, office.
This article first appeared in the September/October 2024 issue of Civil Engineering as “Subterranean Seismic Design.”