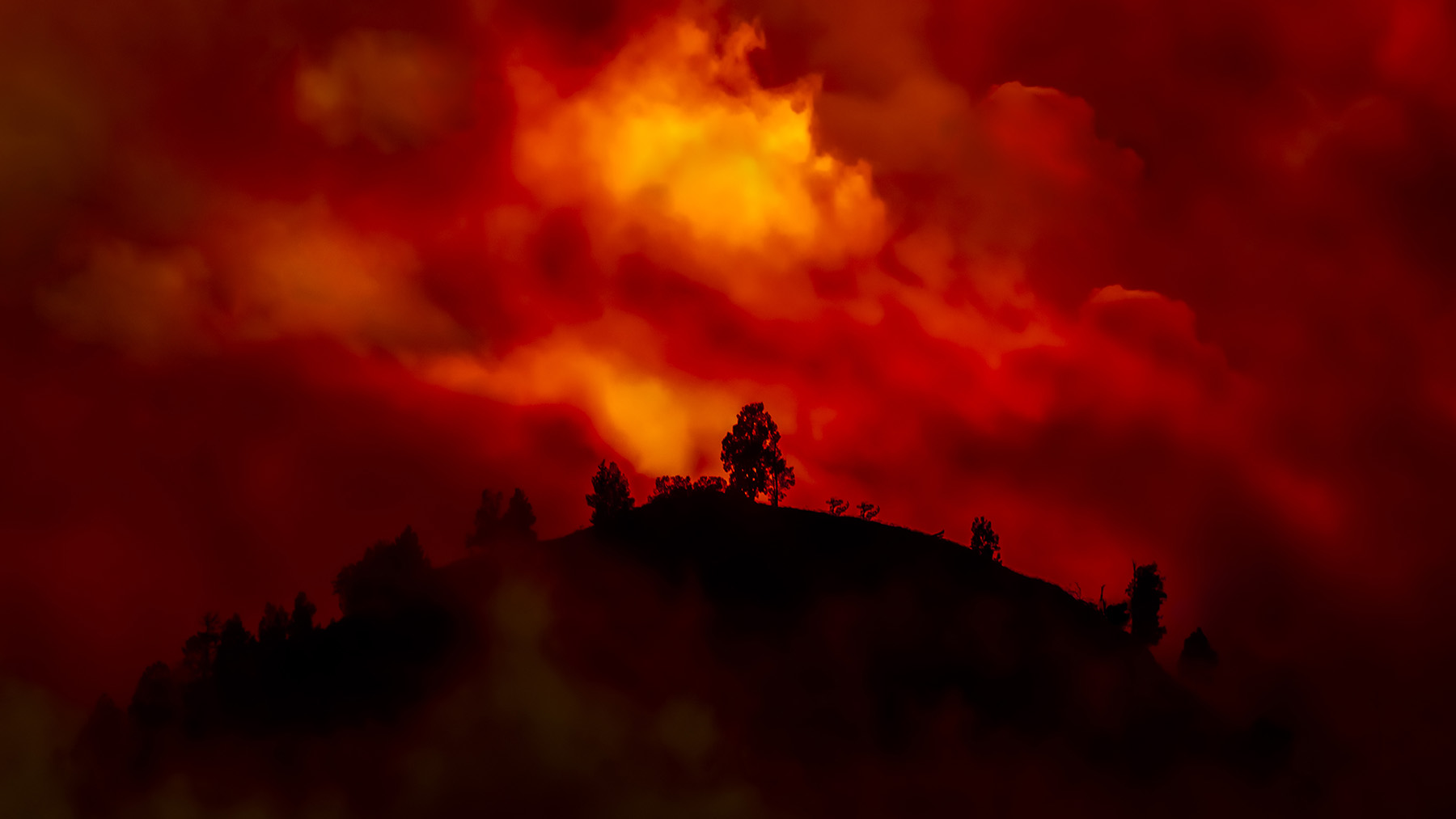
By Robert L. Reid
A warming world is producing more intense wildfires and more extreme heat waves. Here’s what engineering experts are doing to protect and preserve our threatened infrastructure.
The environmental crisis known as climate change has also often been called global warming. That concept of a warming world is critical to understanding the threats posed by wildfires and heat waves to human life, animals, plants, and property, especially the public infrastructure.
The first article in Civil Engineering’s series about making infrastructure more resilient to climate change (“How to make infrastructure more resilient against climate change”) cited the warning from United Nations Secretary-General António Guterres that the world body’s most recent climate report represented “a code red for humanity.” In this second installment, Civil Engineering turns to the proverbial red zone of wildfires and heat waves, contacting more than a dozen civil and structural engineers in private industry, public service, and academia who are experts regarding such events. We also consulted numerous reports, documents, and other resources to highlight the ongoing challenges created by these climate change hazards.
‘Spreading like wildfire’
Globally, the number of extreme wildfires is expected to increase by up to 50% by the end of the century, according to a February 2022 report, Spreading Like Wildfire: The Rising Threat of Extraordinary Landscape Fires, which was prepared by the United Nations Environment Programme and GRID-Arendal, a nonprofit environmental communications center established by the government of Norway. The report also describes the relationship between wildfires and climate change as “mutually exacerbating” because the effects of climate change — including increased droughts, high air temperatures, and other factors — are creating hotter and drier fire seasons that last longer than previously.
At the same time, wildfires can also destroy “sensitive and carbon-rich ecosystems like peatlands and rainforests,” which “turns landscapes into tinderboxes, making it harder to halt rising temperatures,” the report explains.
Wildfires happen on every continent except Antarctica, the report notes, and such fires are “growing in intensity and spreading in range.” In the United States alone, the estimated annualized economic burden caused by wildfires ranges from roughly $71 billion to more than $347 billion, the report states. In most nations, the costs of wildfires have not been assessed, the report adds, but the amount that most governments generally spend related to wildfires is known. Roughly half of these expenditures are for response, and only about 0.2% is spent on planning.
To “reduce the outsized costs from damage and loss,” the report suggests “rebalancing investments.” Although no optimal allocations have been determined, the report considers these amounts to be a good starting point: roughly 34% for response, 32% for prevention, 20% for recovery, 13% for preparedness, and 1% for planning.
An environmental disservice
Ironically, one of the challenges associated with wildfires today — at least in the United States — is that throughout most of the 20th century, the U.S. Forest Service and the Bureau of Land Management tried to fight and extinguish every wildfire that occurred within their jurisdictions, says Erica Fischer, Ph.D., P.E., M.ASCE, an assistant professor of civil and construction engineering at Oregon State University. But toward the end of the last century, scientists began to understand that “wildfires are really beneficial to our landscape — animals and plants actually benefit from the burn,” Fischer says. “So we were doing the environment a disservice by putting out all wildfires.”
Moreover, decades of such “heavy-handed fire-suppression” policies — together with the dryer weather and reduced moisture in vegetation caused by climate change — helped create the conditions by which today’s wildfires burn hotter and with greater intensity than traditionally, and “the risk of wildfire is increasing everywhere,” says Michele Barbato, Ph.D., C.Eng, P.E., F.EMI, F.SEI, F.ASCE, a professor of civil and environmental engineering at the University of California, Davis.
Those fire-suppression policies helped leave much more fuel available for modern wildfires to burn, which results in fires that consume even large trees that might have survived earlier fire events, explains Barbato, who is also a director of the UC Davis Climate Adaptation Research Center and the faculty director of the Climate Initiative of the Center for Information Technology Research in the Interest of Society and the Banatao Institute.
“So now you see wildfires with severities that are burning 90% of everything” in their paths, which “was not the way wildfires used to burn,” Barbato explains. From the standpoint of climate change, the situation has become so out of balance that some forests, especially in California, are no longer carbon sinks. “They’re actually producing more carbon than they can absorb,” Barbato says, which will likely continue for the next decade or two “because the forests need to return to their natural, healthy state before they can again be a carbon sink.”
Add in the fact that more communities are being developed in high-risk fire areas and “the result is increased threat to human life ... and we just can’t keep up with it,” stresses Fischer.
Fortunately, Fischer adds, civil and structural engineers understand how communities function, how infrastructure is impacted by adverse conditions, and how various infrastructure systems intersect. These engineers have long helped communities mitigate for hurricanes, floods, earthquakes, and other disasters or challenges. So now, she concludes, “we need them to start engaging and mitigating against this (wildfire) hazard.”
Triple threat
The most obvious threat to infrastructure from wildfires is the damage and outright destruction caused by the heat and flames. Buildings, bridges, power line systems (structures, conductors, and hardware), and other facilities, especially those made of wood, are all vulnerable to wildfires. But wildfires can also damage underground utilities, contaminate drinking water, and alter the soil chemistry and destroy vegetation at a burned site in ways that increase the risks of flooding or landslides for years after the fire has been put out.
Resilient building design is listed in the Spreading Like Wildfire report as one of many possible actions aimed at reducing the risks posed by wildfires. Regarding structures, those risks manifest themselves in three main ways, notes Ibbi Almufti, P.E., S.E., the West Coast Risk and Resilience leader in the San Francisco office of the global built environment consulting firm Arup.
The first way is by flame impingement, which involves the fire making direct contact with the building, says Almufti, who is also a wildfire mitigation specialist certified by the National Fire Protection Association. The second is through radiative heat transfer during which the flames do not actually touch the structure but do produce enough heat from a distance to ignite the materials on the structure’s exterior. The third method is through airborne embers that can be carried along on the wind — sometimes from a fire more than a mile away. These embers then land either on the structure or on something close enough to the structure (such as nearby vegetation or a combustible fence connected to the structure) so that the embers cause the structure to ignite.
Embers can also enter a structure through external vents or a window that breaks from the heat and ignite the building from the inside out, says Fischer. That sort of ignition is especially challenging because while there are ways to make the exterior of buildings essentially noncombustible, the interiors are almost always filled with combustible finishes and furnishings, Fischer notes.
Almufti recently designed a private residence in California using noncombustible materials on the building envelope to make it more resilient to wildfires. The same principles can easily be applied to various types of public buildings, he adds. Such a structure would feature a metal roof with no valleys or gutters where combustible debris might collect, metal or concrete flashing, and special metal mesh coverings for the louvres or vents to prevent embers from entering the building.
The design would also include a defensible space around the building — based on guidance from the NFPA — of at least 5 ft between the structure and any landscaping. This space — dubbed the immediate zone, as well as the ember-resistant zone — must be clear of any combustible debris, vegetation, flammable materials such as wooden mulch, or combustible attachments that could carry a fire back to the structure, Almufti notes.
Beyond that initial buffer zone, in the 5 to 30 ft area around the structure — called the intermediate zone — vegetation should still be fairly sparse and any trees should be spaced far apart with the limbs kept back at least 10 ft from the building, Almufti says.
Farther out from the structure — in the 30 to 100 ft zone — more vegetation would be acceptable, though still somewhat limited. The distance between trees here should also be controlled “so you don’t have a canopy fire that transfers across the crowns of the trees,” Almufti adds.
Building bricks better
One potential material for making buildings more resilient to wildfires has a history that dates back 10,000 years or so, says Barbato. He is referring to mud bricks, which have been used as a building material since ancient times. Barbato’s research actually involves a modern, engineered form of mud bricks called compressed and stabilized earth blocks.
Although originally considered for resilience against such hazards as earthquakes, hurricanes, and tornadoes, the earth blocks have also been tested in furnaces at temperatures of nearly 2,200 F — without burning. In fact, Barbato notes, “structurally, when you subject these bricks to severe fire like in wildfires, the material gets stronger.” During one test with a compression machine, for example, the test ultimately had to be called off because the machine simply could not break the fire-strengthened bricks, he explains.
So far, the bricks have been used primarily in high-priced private homes and for the construction of small houses to help address homelessness, Barbato says. And there is a potential limitation on the height of buildings constructed with the bricks because as the building height increases, the walls of bricks need to become thicker to provide the same resistance factors. But the bricks work quite well with low-rise buildings of one or two stories, he notes.
Artistic expression
For Thomas Sabol, Ph.D., P.E., S.E., a principal at the structural engineering firm Englekirk, in Los Angeles, the city’s Getty Center — an art museum, research and conservation center, and home to the Getty Foundation — represents “the most deliberate project for addressing” the hazards of wildfires that he has ever worked on. Opened in 1997, the Getty Center famously survived wildfires in 2017 and 2019, the latter event named for the site, without having to evacuate its art treasures.
The Getty Center, which describes itself as a “marvel of anti-fire engineering,” was designed by architect Richard Meier and Englekirk to safeguard the facility with features that range from the landscaping and roadways to the construction materials and ventilation systems to key structural elements within and even under the building itself.
The complex’s multiple buildings and grounds feature fire-resistant stone, concrete, and steel with large, open spaces designed to slow a spreading fire, explains an Oct. 29, 2019, blog post, “Why the Getty Center Is the Safest Place for Art During a Fire.” The landscaping is kept well irrigated due in part to a network of underground irrigation pipes and a 1 million gal. subterranean water storage tank beneath the center’s main building, says Sabol. Intended to provide an independent source of firefighting water in case the site’s access to the public waterlines is interrupted, the tank features interior baffles to control sloshing.
The main building is also divided into two independent structures by a large wall of reinforced concrete and concrete blocks known as the maximum foreseeable loss wall. A requirement of the center’s insurance provider, the MFL wall separates the museum galleries on the south side of the building from the offices, auditorium, cafe, and other functions on the north side. It is intended to provide four hours of fire protection and prevent one side of the building from collapsing should the other side succumb to a fire, Sabol explains.
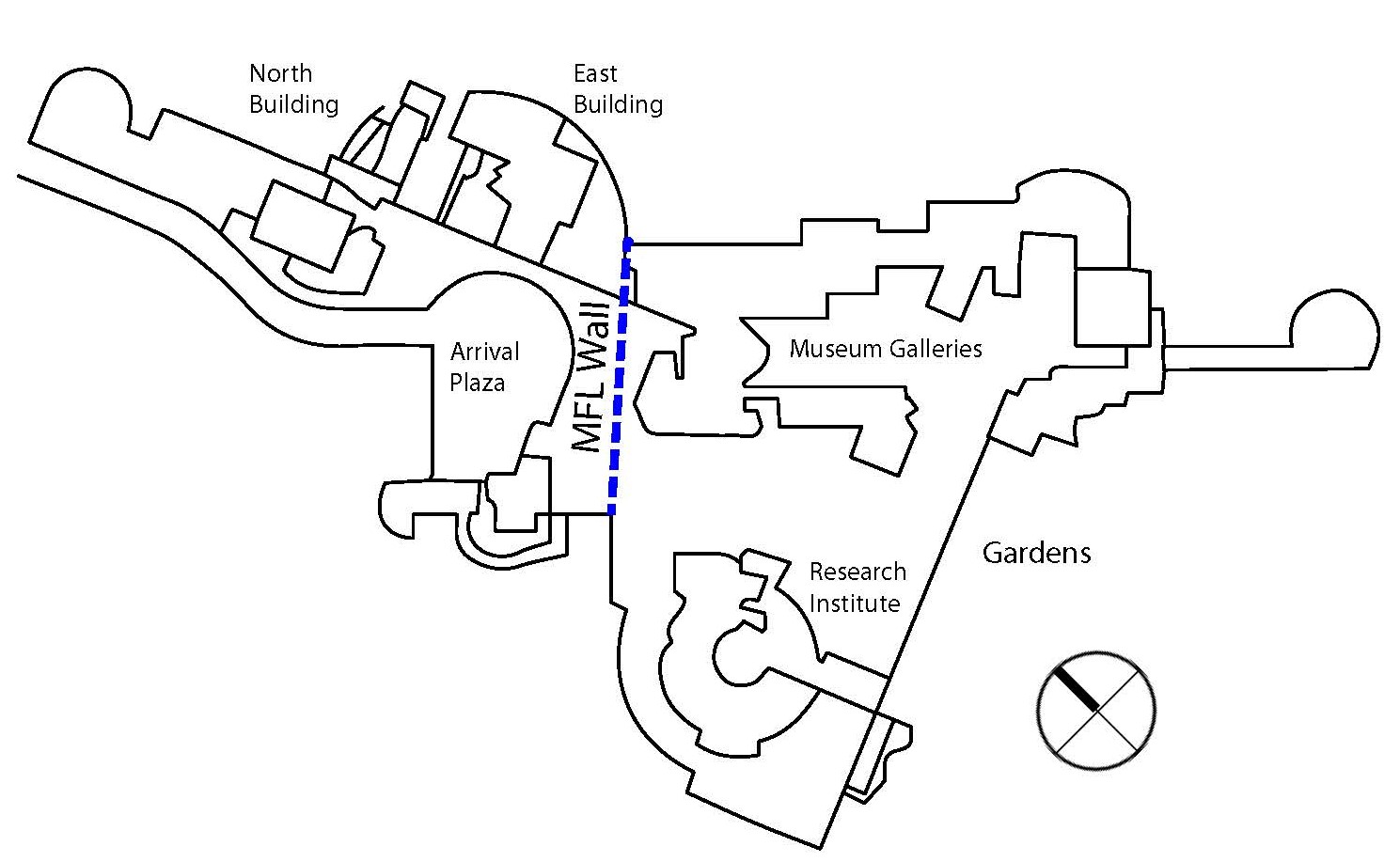
Other aspects of the facility’s fire resilience include a road network and ramp that enable firefighting vehicles to easily access the building, which, because of the site’s steep slopes, might otherwise be inaccessible, Sabol says. Because the site is subject to landslides, the road system required various robust retaining structures to support the soil and the surcharge imposed by the firefighting vehicles. Large retaining walls were also required to support a roughly 30 ft diameter circular green space known as the North Promontory that can accommodate the weight of a helicopter, in case firefighters can only arrive by air or an aerial evacuation is required.
The Getty Center also features fire-resistant folding divider walls that can seal off sections of the museum, a pressurized air system to keep out smoke, fire-resistant crushed stone on the roofs of the buildings, a buffer zone without vegetation around the buildings, and regular pruning of the site’s oak trees to ensure that their canopies remain high off the ground, according to a Dec. 12, 2017, New York Times article, “Why the Getty Center’s Art Stayed Put as Fires Raged Nearby.”
Double-edged sword
The 2019 Getty Fire was reportedly started by a tree branch hitting a power line. The threat posed by the proximity of vegetation to electrical infrastructure is part of a “double-edged sword” that electrical utilities face from such events, notes Otto Lynch, P.E., F.SEI, F.ASCE, a vice president of Bentley Systems and the head of Power Line Systems, Lynch’s Madison, Wisconsin-based firm that was recently acquired by Bentley.
Electrical utility infrastructure systems are both vulnerable to damage caused by wildfires and potentially the cause of those same wildfires, Lynch explains. Many utilities predominantly use wooden poles for their distribution lines that carry power directly to the customers and the smaller transmission lines that deliver power to the distribution substations. These wooden poles are more prone to damage from wildfires.
The larger transmission towers that carry the highest-voltage power lines from generating stations to electrical substations are generally made of steel and are often considered less vulnerable. When exposed to high heat, however, even the material properties of steel can weaken and fail, says Clinton Char, P.E., M.ASCE, the manager of the transmission engineering structural group at Southern California Edison, which provides power to incorporated cities and counties throughout the southern and central parts of the Golden State. SCE has begun to examine this phenomenon with Matergenics Inc., a Pittsburgh-based metallurgical engineering company, Char adds.
To help obviate the threat posed by vegetation and power lines, utilities need to view their systems three-dimensionally, Lynch notes. Too often, the clearances of vegetation and power lines are examined during so-called average days with no wind and average electrical loadings. But the utilities actually need to know how the wires will be blowing in the middle of a storm. This is especially true when conductor temperatures have increased due to hot weather, and high electrical loads have caused the lines to sag significantly more, Lynch says.
Determining where the lines will be during these critical events can help a utility proactively trim the trees or other vegetation in the most vulnerable areas. This also results in more targeted and selective vegetation management, rather than just cutting back everything that is within the right of way, which can be controversial and risk public opposition, Lynch adds.
Burying distribution power lines underground is also a solution, especially for new developments. But it is extremely expensive — as much as 10 times or even 20 times the cost of overhead lines, which, of course, the customers eventually end up paying for, Lynch says.
Utility improvements
At SCE, the instances and severity of wildfires have been increasing for at least the last decade, says Char. SCE’s distribution system features some 1.2 million wooden poles, many of them in grasslands and forested areas that are prone to wildfires due to the long, dry summers the region has been experiencing because of climate change.
Having lost a “significant” number of wooden poles to wildfires over the past several years, SCE has been replacing those vulnerable structures with poles made from other materials, including steel and fiberglass, Char says. The utility has also been testing various protective coatings or shields that can be wrapped around wooden poles. These include protective systems that can be incorporated into a new pole during the manufacturing process as well as systems that can be retrofitted to existing poles.
Such protected poles have been installed within SCE’s service area and have performed quite well, having survived actual fires, Char adds.
SCE generally runs the electricity through “bare” wire conductors, which are made from either aluminum, aluminum alloy, or copper and have no insulation or other protective coatings. During high-wind events, if a tree branch or any sort of combustible debris makes contact with the conductor, sparks could be generated, which could start a wildfire if there is dry brush in the area, Char explains.
To protect against such incidents, SCE has been replacing bare conductors with covered conductors — insulated or coated wires — in high-fire areas. They minimize the potential for spark generation and wildfire initiation during high-wind events, Char says.
Although it is hard to determine how effective the approach has been, “we have had wind events in high-fire areas over the past few years, and we have noticed there have been lower incidences of wildfires starting in those areas,” Char notes.
Shutting off power
Like other utilities in its region, SCE has adopted a strategy called Public Safety Power Shutoff, in which the utility will proactively turn off power in areas experiencing high winds to minimize the chance of a power line causing a fire. Not surprisingly, turning off power to communities is a contentious and sensitive issue, Char says, so the utility is also using meteorological data, complex computer models, and other factors to better determine the wind speeds its system can accommodate.
Although SCE often relies on National Weather Service information, which is often collected at airports or other locations and then must be interpolated for the specific sites of the utility’s infrastructure, the firm has also been installing weather-monitoring equipment “right at our lines so we know what the exact conditions are at our particular sites,” Char says.
In addition, SCE is conducting pilot studies on microgrid systems that could be isolated from the overall power system so that if the utility needs to shut off power in some areas, microgrid sections might still have power.
Metro moves
For the Los Angeles County Metropolitan Transportation Authority, wildfires as well as heat waves present more of an indirect threat than an immediate danger, says Cris Liban, D.Env., P.E., ENV SP, Dist.M.ASCE, the L.A. Metro’s chief sustainability officer. By that, Liban means there is a much greater chance that the transportation system will lose electrical power because of a wildfire or heat wave — from fire- or heat-related damage to the electrical grid infrastructure or a proactive power shut-off — than there is that the flames from a wildfire will actually burn the trains or buses that L.A. Metro operates.
In 2020, L.A. Metro received about 32% of its power from SCE and 63% from the Los Angeles Department of Water and Power. The agency is working with utilities to have its rail system designated at the highest priority to avoid power shut-offs, Liban notes. L.A. Metro is also exploring distributed energy systems and working with local clean and renewable energy providers — even considering deals under which it would become the sole customer of new green energy sources — to help ensure it receives the power it needs, Liban says.
After the fire
Some of the greatest challenges from wildfires do not manifest themselves until after the fires have been extinguished. A wildfire might make a road temporarily impassable, but the road itself can remain intact after the fire passes by, says Mikhail Chester, Ph.D., A.M.ASCE, an associate professor of civil, environmental, and sustainable engineering at Arizona State University.
But the fire can cause secondary effects. “After the wildfire, lots of things have changed,” Chester explains. “The soil chemistry and vegetative conditions have changed” at the burned site. For example, the soil might be much more hydrophobic, meaning it will not absorb water as well as it did prior to the fire. And there could be lots of debris on the ground. Together, those factors could generate a post-wildfire debris flow during subsequent rain events, even relatively mild rains.
“These debris flows could be hundreds of times more intense than had the same rain fallen prior to the wildfire going through that area,” Chester explains. The result could be an overtopping of a road, making it impassable again, or a culvert or channel for stormwater could be damaged. The road could be eroded or the subbase compromised, Chester notes.
Wildfires can also cause contamination to underground water pipe networks, notes Fischer. The sources of this contamination can be from debris, soot, and ash sucked into the distribution system because of negative water pressure created as firefighters and homeowners try to put out the blazes.
Thermal degradation of plastics installed within the distribution system is another possibility. Following the November 2018 Camp Fire in Paradise, California, and surrounding areas, a response team from Purdue University and Manhattan College investigated the damage sustained by Paradise’s buried drinking water infrastructure and discovered contamination from chemicals such as benzene, toluene, dichloromethane, and styrene at levels above the state or federal safe drinking water limits (read “Fire & Water,” Civil Engineering, January/February 2021, pages 42-47).
Thermal degradation of plastics installed within the distribution system is another possibility. Following the November 2018 Camp Fire in Paradise, California, and surrounding areas, a response team from Purdue University and Manhattan College investigated the damage sustained by Paradise’s buried drinking water infrastructure and discovered contamination from chemicals such as benzene, toluene, dichloromethane, and styrene at levels above the state or federal safe drinking water limits (read “Response team investigates wildfire damage to buried drinking water infrastructure,”).
To help make communities more resilient to post-fire drinking water contamination, Fischer and her Oregon State colleagues have been conducting heat transfer experiments on pipes to determine what levels of contamination might result from exposure to various temperatures. They have also collaborated closely with Lauren Linderman, Ph.D., EIT, A.M.ASCE, an associate professor of civil, environmental, and geo-engineering at the University of Minnesota, who is developing sensors that can identify the areas at greatest risk of water contamination based on how close the pipes come to a threshold temperature during the fire, says Fischer.
Currently, communities must dig up potentially hundreds of miles of pipes to determine their conditions after a fire, Fischer explains. “But if you had sensors on the pipes, you’d be able to say, ‘This pipe we have to take out but not that pipe over there.’” The sensors would enable communities to target their responses, saving time, energy, and effort.
Communities can also try to develop databases of what materials were used in which pipes within their systems so that after a fire they can “understand where the contamination might come from ... so they can respond in a strategic and targeted way,” Fischer says.
Smoke is also a major concern from wildfires — and one that might lead to certain trade-offs among the various responses to climate change. Naturally ventilated buildings, for example, have been touted as a measure to help mitigate climate change rather than relying on greenhouse-gas-emitting air-conditioning equipment. But during wildfires, air conditioning or air purification is likely necessary, at least in a hybrid approach that lets the building use natural ventilation when environmental conditions are conducive but which also features a standard air-conditioning system triggered by heat or air quality, says Alexej Goehring, P.E., an Arup associate principal and the firm’s building environmental and physics leader for Arup in the Americas.
A warming world
As the world grows warmer, the impact of heat waves is being felt across the nation. More than a decade ago, heat waves from one end of the country to the other caused widespread challenges, according to Flooded Bus Barns and Buckled Rails: Public Transportation and Climate Change Adaptation, an August 2011 report from the Federal Transit Administration.
Excessive heat damaged the rails of commuter train systems in Washington, D.C., and Boston; stretched catenary systems, disrupting the power to rail lines in New Jersey and California; and caused electronic control systems for trains to overheat in Portland, Oregon, where the climate had been “historically mild,” the FTA report notes. Extreme heat can also “stress materials, reduce asset life, and jeopardize customer and worker health and safety,” the report concludes.
In 2012, a jet airliner at Ronald Reagan Washington National Airport, outside the nation’s capital, was briefly stuck on the tarmac because a wheel sank into the softened surface following several days with temperatures above 90 F.
Just last year, record high temperatures in Portland — reaching at least 115 F — melted power cables, suspending streetcars and light-rail service, while roads and pavements began to soften and buckle.
In October 2021, the city of Phoenix established a new Office of Heat Response & Mitigation, and in April the U.S. Department of Labor’s Occupational Safety and Health Administration announced its first-ever national emphasis program “to protect millions of workers from heat illness and injuries.” Noting that “the three-year average of workplace deaths caused by heat has doubled since the early 1990s,” OSHA stressed that it is “continuing long-term work to establish a heat illness prevention rule.”
In October 2021, the city of Phoenix established a new Office of Heat Response & Mitigation, and in April the U.S. Department of Labor’s Occupational Safety and Health Administration announced its first-ever national emphasis program “to protect millions of workers from heat illness and injuries.” Noting that “the three-year average of workplace deaths caused by heat has doubled since the early 1990s,” OSHA stressed that it is “continuing long-term work to establish a heat illness prevention rule.”
Urban experience
Heat waves can be especially challenging within cities, where pavement and buildings increasingly replace open land and vegetation, reducing shade and making urban areas warmer than surrounding rural zones. “Surfaces that were once permeable and moist generally become impermeable and dry,” according to the U.S. Environmental Protection Agency’s document, Reducing Urban Heat Islands: Compendium of Strategies, originally written in 2008 and updated in 2012. In a city, exposed surfaces like roofs and pavement can reach temperatures as much as 90 F hotter than the surrounding air, explains the report.
Consequently, cities are trying to increase their resilience to heat waves by planting more trees and/or other vegetation as well as installing shade structures and applying special coatings to roofs and pavement that better reflect sunlight, reducing the amount of heat those surfaces absorb.
To make transit systems more resilient to the effects of heat waves, for example, engineers have helped design approaches that range from installing expansion joints in rail lines to reduce track buckling to incorporating solar-powered cooling systems at transit stops. Engineers are even examining satellite images to identify the hot spots within a city where the temperatures might be the most severe and thus where measures such as increasing the urban tree canopy might be the most effective, according to the FTA report.
In Cambridge, Massachusetts, the Department of Public Works coated a city-owned parking lot with a reflective paint roughly the color of sandstone that helped reduce the lot’s surface temperature by as much as 15 F compared with a typical asphalt surface on an 89 F day in full sunlight, explains Kyle Johnson, a climate resiliency specialist, and Ariel Patterson, a resiliency planner, with the engineering firm Kleinfelder. The city is working with Kleinfelder to monitor surface and ambient air temperatures for the project.
Cambridge also incorporated high-albedo pavers and artificial shade structures in public parks and open spaces. A district-scale stormwater project in one neighborhood is also maximizing future tree canopy as a means of addressing urban heat island effects, and the city passed an ordinance in May 2021 that requires green roofs on new buildings larger than 25,000 sq ft, according to Kleinfelder’s team.
Likewise, a “cool pavements” program in eight Phoenix neighborhoods helped reduce average surface temperatures at noon by as much as 12 F compared with sites with traditional asphalt, according to a September 2021 announcement from the city’s Street Transportation Department and Office of Sustainability.
L.A. Metro is working with regional stakeholders to add canopies for shade at key bus stops, sometimes using new types of light-reflecting materials and designs that his agency’s engineers might not be familiar with, notes Liban. So special training can be required to help engineers understand and maintain such systems, align them properly, and even keep them clean, he explains.
Heat effects
Over the past decade, Seattle has experienced an increasing number of excessively hot days, with temperatures in the 90 F range and even above 100 F at times. This has led the city to temporarily close its movable steel bridges at times so that a truck can spray water along the structures to cool them down. The water is meant to prevent the bridges’ steel elements from swelling in the heat and locking up, explains Kit Loo, P.E., a bridge operations and engineering manager with the Seattle Department of Transportation.
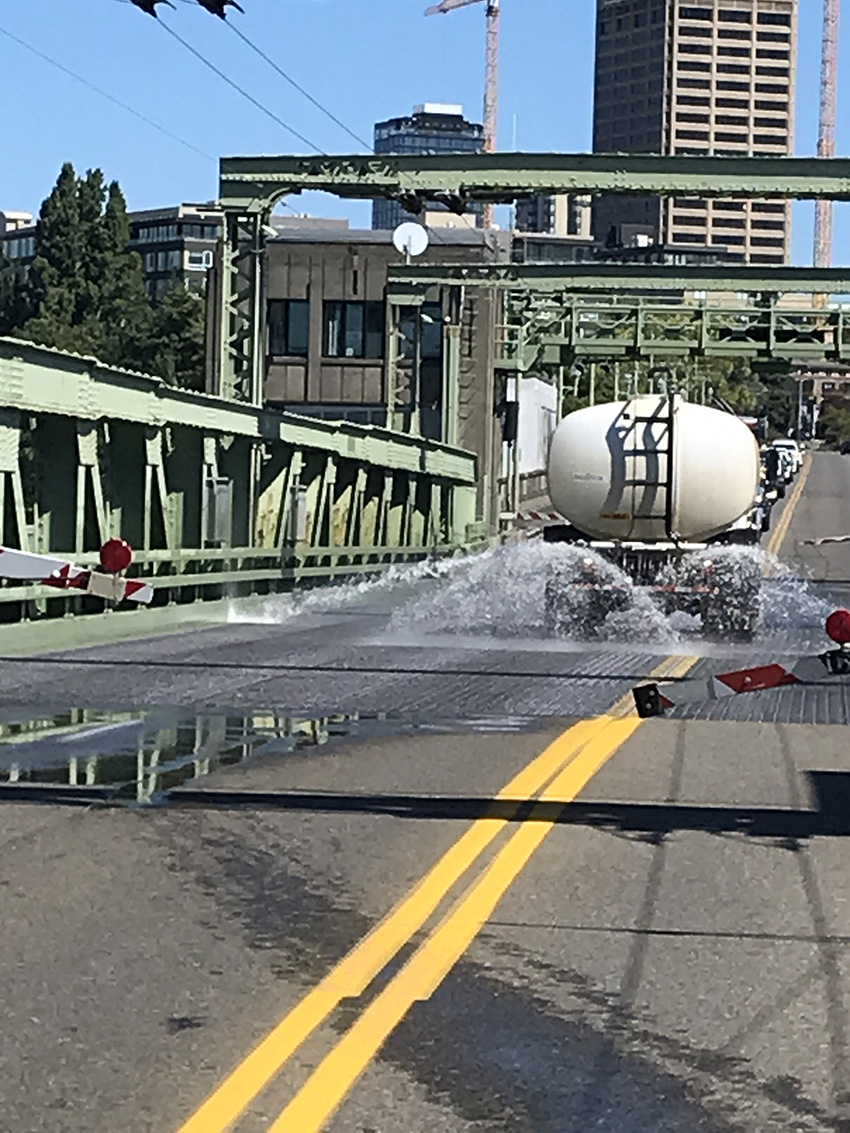
Called a flusher truck, the vehicle is generally sent out before the hottest period of the day to proactively keep the bridges cool, Loo says. It is then sent out again on an hourly basis as needed. Bridge operators use handheld infrared thermometers to track the temperatures of the bridges’ steel.
Chicago has had similar experiences, although there the city cools bridges with water sprayed from a fireboat on the Chicago River.
Excessive heat will hinder the performance and efficiency of almost any infrastructure system, notes Chester. Power lines, water pumps, and other equipment are engineered to give off heat as they operate. But when the surrounding air is excessively hot, these systems cannot get rid of the heat they generate as quickly and as easily as on cooler days. So they have to work harder to provide the same service — whether it’s generating electricity or moving water through pipes — which leads to more frequent failures. And given the fact that most infrastructure is currently underfunded, even a small increase in failures can become a large problem, Chester says.
Knowing what to do
Information is a key tool for engineers trying to improve the resilience of infrastructure, whether the data are gained from sensors on buried water pipes or temperature gauges on steel bridges. “I can’t tell a community what to do,” explains Fischer, “but I can give them information so they can make an informed decision that’s right for them.”
At Carnegie Mellon University, civil and environmental engineering professors Mario Berges, Ph.D., A.M.ASCE, and Matteo Pozzi, Ph.D., A.M.ASCE, are improving computer models so that they integrate temperature predictions with energy consumption in order to assess the risk from problems induced by extreme heat.
“We hope (these models) will help better optimize the use of resources,” says Pozzi, so that cities can make better decisions regarding what sources of energy to use, where to locate cooling centers during heat waves, or when and where to issue warnings about expected extreme conditions, especially given the potential differences in temperatures caused by the presence of vegetation in a particular area, the proximity to water, or even elevation and wind conditions.
Berges concludes: “We will be more resilient the better prepared we are with models about how heat influences infrastructure.”
Robert L. Reid is the senior editor and features manager of Civil Engineering.
This article first appeared in the July/August 2022 issue of Civil Engineering as “The Red Zone.”