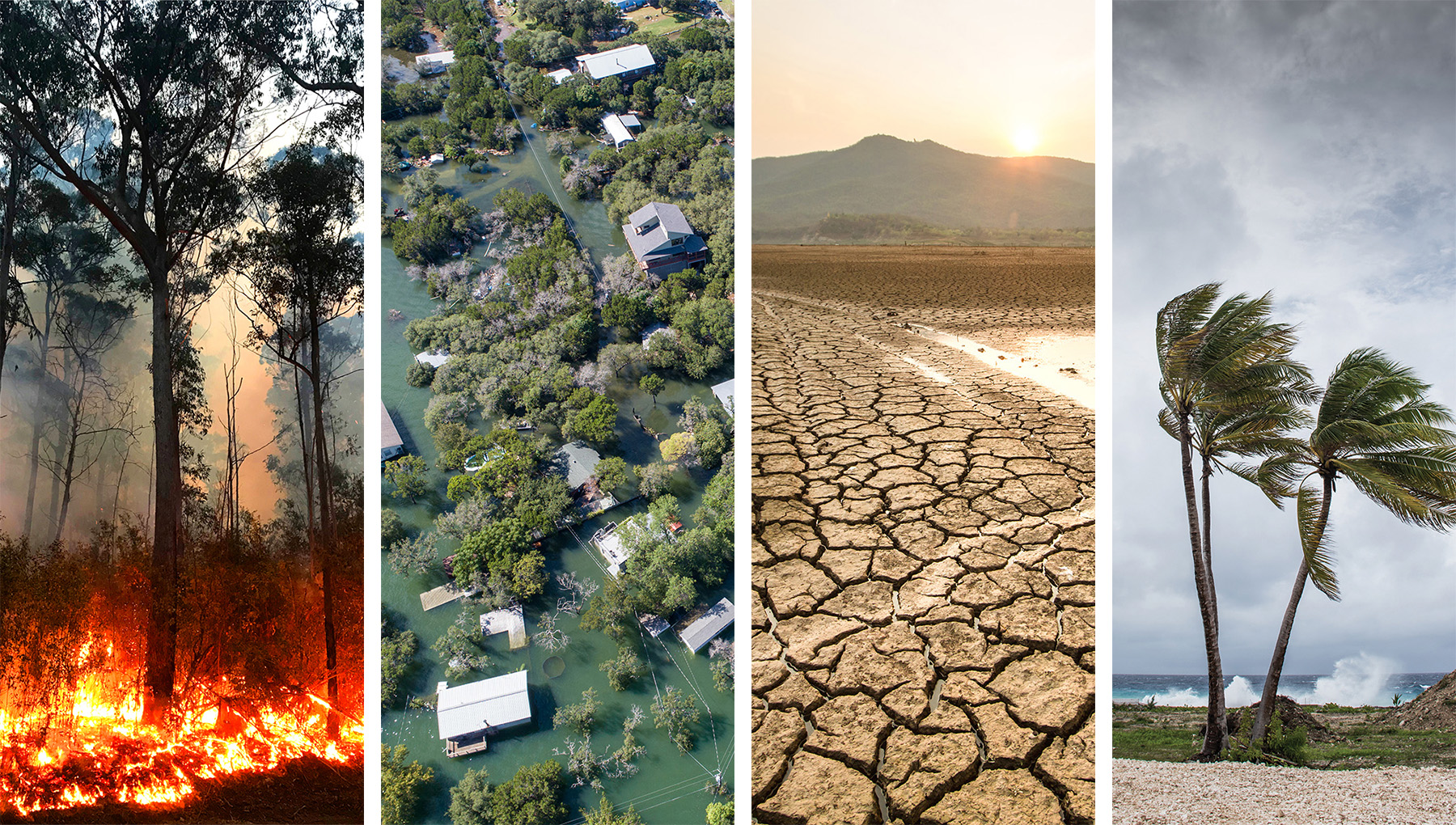
By Robert L. Reid
United Nations Secretary-General António Guterres describes the latest Intergovernmental Panel on Climate Change report on global warming as “a code red for humanity.” Civil engineers are working to limit the emission of greenhouse gases, preserve resources, and promote resiliency to ensure that the infrastructure they design and build today will withstand the impacts of a changing environment tomorrow.
This is the first in a series of articles on infrastructure resilience that Civil Engineering plans to publish this year. Future articles will focus on specific climate threats and how civil engineers are working to mitigate the potential damage and keep critical infrastructure systems in operation.
The world’s climate is changing — and those changes are bringing significant impacts that will affect the way infrastructure is designed and constructed. Civil Engineering spoke with more than a dozen engineers — from the public and private sectors, academia, and advocacy groups — who are involved in studying the potential impacts of climate change and working to develop the proper engineering responses. They make it clear that the civil engineering profession has a significant role to play in helping the world adapt to these new conditions.
On Aug. 9, 2021, the United Nations’ Intergovernmental Panel on Climate Change released its latest report — Climate Change 2021: The Physical Science Basis — warning that climate change “is widespread, rapid, and intensifying.” Prepared by 234 scientists from 66 countries, the IPCC report declared that human-induced climate change “is already affecting many weather and climate extremes in every region across the globe ... in the atmosphere, in the oceans, (in) ice floes, and on land.”
Many of the observed changes “are unprecedented,” the report states, “and some of the shifts are in motion now, while some — such as continued sea level rise — are already ‘irreversible’ for centuries to millennia ahead.”
U.N. Secretary-General António Guterres described the report as “a code red for humanity,” adding that the “alarm bells are deafening, and the evidence is irrefutable.”
The report’s findings included:
- In 2019, concentrations of atmospheric carbon dioxide — one of several greenhouse gases that is contributing to a warming globe — were higher than at any time in at least 2 million years. Concentrations of methane and nitrous oxide, two other important GHGs, were higher than at any time in the last 800,000 years.
- The global surface temperature has increased faster since 1970 than in any other 50-year period over at least the last 2,000 years.
- The global mean sea level has risen faster since 1900 than over any preceding century in at least the last 3,000 years.
- By 2021, emissions of GHGs from human activities were responsible for approximately 1.2 degrees Celsius of warming above preindustrial levels. Averaged over the next 20 years, the increase in global temperature is expected to reach or exceed 1.5 degrees Celsius.
“Science has long warned that we need to limit temperature rise to 1.5 degrees” above preindustrial levels, Guterres stressed. But that threshold is coming “perilously close,” and if it is exceeded “we risk calamity,” he warned.
Feeling the impact
The world is already experiencing the effects of climate change, the report noted, in the form of prolonged droughts, increased numbers of wildfires, extremes in precipitation, extensive flooding, intense tropical cyclones, and the loss of Arctic sea ice, snow cover, and permafrost. Such effects will likely increase if action is not taken to limit warming.
At 1.5 degrees Celsius of global warming, there will be “increasing heat waves, longer warm seasons, and shorter cold seasons,” the report concluded.
At 2 degrees Celsius of global warming, “heat extremes are more likely to reach critical tolerance thresholds for agriculture and health,” the report stated. Rainfall patterns will also be disrupted, with “more intense rainfall and associated flooding, as well as more intense drought in many regions.”
The effects of climate change “may be magnified” in the world’s cities, especially coastal cities, which could face increasing heat and heavy precipitation, the report noted. “Moreover, coastal areas will see continued sea level rise throughout the 21st century, contributing to more frequent and severe coastal flooding in low-lying areas and coastal erosion,” the report warned. “Extreme sea level events that previously occurred once in 100 years could happen every year by the end of this century.”
Engineering a response
In ASCE’s Policy Statement 418, The Role of the Civil Engineer in Sustainable Development, the Society defines sustainability as “a set of economic, environmental, and social conditions in which all of society has the capacity and opportunity to maintain and improve its quality of life indefinitely without degrading the quantity, quality, or the availability of economic, environmental, and social resources. Sustainable development is the application of these resources to enhance the safety, welfare, and quality of life for all of society.”
In Policy Statement 518, Unified Definitions for Critical Infrastructure Resilience, ASCE defines resilience as the “ability to plan, prepare for, mitigate, and adapt to changing conditions from hazards to enable rapid recovery of physical, social, economic, and ecological infrastructure. Improving resilience before or following a hazard event should engage physical infrastructure and social systems with adaptive capacity to ensure rapid return to functionality, accounting for interdependencies within and across all sectors.”
There is considerable overlap between resilience and sustainability, “and whenever we can synergize them, we need to do so,” says Craig Davis, Ph.D., P.E., G.E., M.ASCE, who recently retired as the chief resilience officer of the Los Angeles Department of Water and Power and now runs his own consultancy, C.A. Davis Engineering, in Santa Clarita, California. But Davis also sees a crucial difference between the concepts: “Sustainability does not include the ability to respond and recover, but resilience does not include the ability for future generations to have resources.”
Caroline Field, P.E., CEng, M.ASCE, an associate director in the Bristol, England, United Kingdom, office of the international engineering firm Arup, notes: “Quite often I hear people say resilience is a subset of sustainability, or sustainability is a subset of resilience. But neither is true — they are different things, but you need them both.”
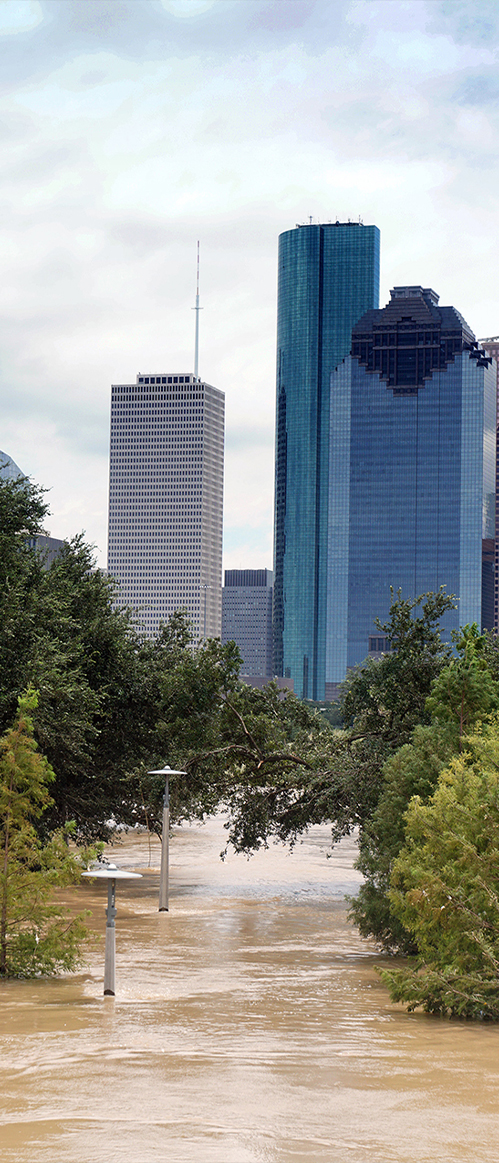
Field also serves as the head of energy for the Resilience Shift — a global nonprofit organization created by London-based Lloyd’s Register and Arup. She is also a Royal Academy of Engineering visiting professor of structure and infrastructure resilience at Loughborough University. She explains that resilience is “more a process for enabling your strategic goals, to make sure you’re prepared for and not disrupted by any challenges ... whereas sustainability is about ensuring we meet the needs of the present without compromising the ability of future generations to meet their own needs.”
With respect to climate change, resiliency is the ability of an infrastructure system to adapt to and withstand various climate-related stressors — which may include the aforementioned storms, floods, droughts, wildfires, etc. — while either remaining in operation or quickly returning to operation after an event, explains Bilal M. Ayyub, Ph.D., P.E., F.SEI, Dist.M.ASCE, a professor of civil and environmental engineering at the A. James Clark School of Engineering at the University of Maryland and the director of the university’s Center for Technology and Systems Management. Resilience is driven by a concern for safety, Ayyub adds, and a resilient design strategy will include the recovery period following a hazardous event.
Ayyub is a co-author of a recent task group report from the ASCE Committee on Sustainability — A Call to Action: Climate Change and Our Uncertain Future, an Opportunity for Commitment and Leadership, which the committee hopes to publish soon. In a draft of the report shared with Civil Engineering, the authors stress: “Each year governments and the private sector invest trillions of dollars in infrastructure that may not withstand future impacts from climate change. But the risks associated with potentially severe future climate events go beyond the economic costs. The changing climate also jeopardizes food and water supplies, damages ecosystems resulting in a loss of biodiversity, and adversely affects our quality of life often on a national and global level.”
To address these challenges, the report adds, “the skills and expertise of engineers will be in great demand. Engineers will work with other infrastructure professionals and project owners to ensure infrastructure is safe, resilient and sustainable and to help meet net zero carbon emissions goals.”
Ayyub also edited two resources that can assist civil engineers as they approach these goals: ASCE’s 2018 Manual of Practice 140, Climate-Resilient Infrastructure: Adaptive Design and Risk Management, and ASCE’s 2021 Manual of Practice 144, Hazard-Resilient Infrastructure: Analysis and Design.
Infrastructure has long been designed to be resilient — but only to those events that are likely to be similar to those in the past and that have therefore been well researched and quantified, such as earthquakes or temperature swings. The field of earthquake engineering, for example, grew from the idea that a building “should not collapse during even the biggest credible earthquake,” says Larry Roth, P.E., G.E., ENV SP, D.GE, D.WRE, F.ASCE, a former deputy executive director at ASCE who recently retired as a vice president of the international engineering firm Arcadis. “The building might be uninhabitable” following an earthquake, explains Roth, who also co-authored the Call to Action report. “It might need extensive repairs — but it should not collapse outright, trapping and killing people inside.”
That approach to resilience must move from earthquake engineering “into more of a general design goal,” Roth says. The idea, he says, is that “if the infrastructure system is stressed beyond what would normally be considered its range of design, it might not function as well as it did before the event, but it won’t collapse,” or otherwise be catastrophically destroyed. “It won’t cause loss of life.” Following the stressor event, the infrastructure should be repairable.
Staying in service
The goal of resilience is to keep critical infrastructure systems operating throughout a hazardous event or, if that is not possible, to minimize any service disruption to a period of time that is “tolerable” to the local community, notes Davis. What is tolerable depends on the community, but to him it means that the infrastructure system must be able to provide its service “when the customers need that service.”
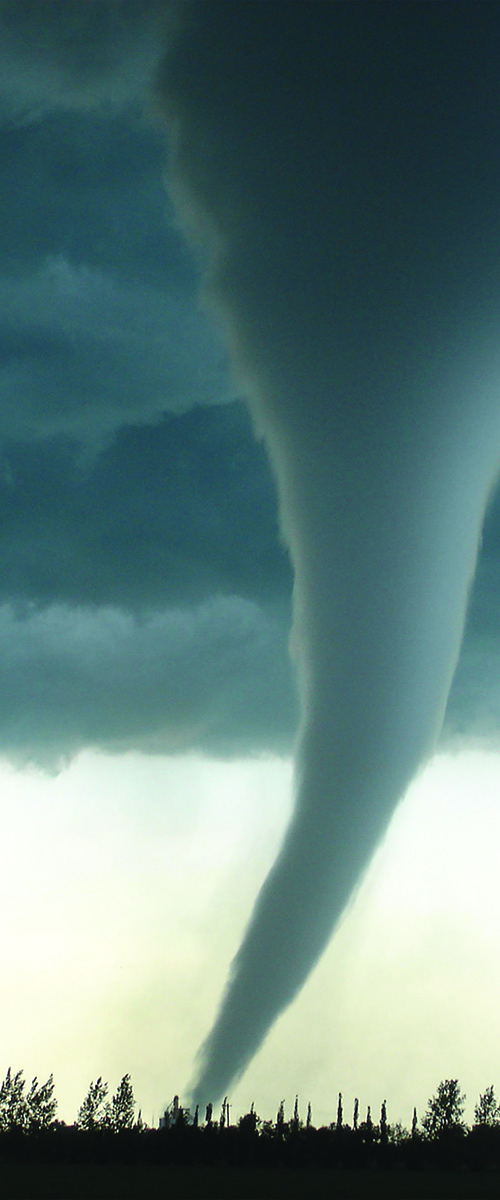
How long that downtime lasts and remains tolerable depends on the community’s access to alternatives and which type of infrastructure system is involved, Davis adds. It may sound harsh, he says, but “embedded in this definition is the idea that not all customers for lifeline systems are equal in a disaster.” For example, firefighters may need priority access to water immediately after an event to fight any fires that may result. And while people obviously need potable water, they will survive if there is no water service for a few hours or even a day or two after an event. It takes at least three days without drinking water before the issue becomes a health concern, Davis explains.
Likewise, it is more important for a hospital, fire station, or police station to remain in operation than it is for some local businesses, Davis says. If the local bowling alley or movie theater loses power, it is an inconvenience and a potential economic problem if those facilities remain closed for too long. But it is a crisis if the hospital, fire station, or police station cannot function.
Davis also stresses that reliability is not the same thing as resilience. “You need reliability to be resilient,” he says, “but reliability alone is not enough.” For example, if workers who need to be on-site to operate a power station cannot get to it because a bridge is out, then the power station cannot provide its service — even if the facility itself is able to function. As could easily have happened during the COVID-19 pandemic, resilience can be compromised even without any damage to physical infrastructure “if a disease keeps people from getting to work,” Davis says.
Entering uncharted territory
Climate change forces civil engineers to work in “uncharted territory,” says William A. Wallace, ENV SP, F.ASCE, a former senior vice president at CH2M Hill (now part of Jacobs) and recently retired from the Wallace Futures Group, which he founded in Steamboat Springs, Colorado. Wallace also co-authored the Call to Action report. Wallace cites the work of American environmental activist and author William McKibben, who wrote that the Earth has enjoyed a kind of climate equilibrium for the last 10,000 years or so, with average temperatures of roughly 14 to 15 degrees Celsius, predictable rainfall, and seas that were relatively tame and level. Weather conditions might vary day to day, but the overall climate was statistically constant — a condition called stationarity, Wallace says.
As a result of that climate stationarity, civil engineers could use historical climate conditions to predict future conditions. This made it possible to design, build, and operate long-lasting infrastructure systems, “confident that they will be safe, reliable, and functional throughout their design life,” Wallace says. Such systems were designed to accommodate the most likely climate conditions with added “factors of safety to handle the occasional extreme conditions.”
This assumption of stationarity was “baked into the codes and standards civil engineers use to design, build, and operate civil infrastructure,” Wallace adds.
But if the climate is no longer stable, “then stationarity is no longer a valid assumption,” Wallace says. “And the codes and standards are no longer reliable.” Extreme weather conditions that were once considered improbable “have become more probable,” he says, which “means that infrastructure failures are more likely to occur.”
Facing such extreme conditions, civil engineers tend to respond by strengthening or otherwise trying to protect infrastructure assets from damage, Wallace notes. And while such an approach “still needs to be part of the engineer’s toolkit,” he says, “the fact that they are in uncharted territory calls for new thinking.”
“It’s possible for a piece of infrastructure to meet all the tests for resiliency” in a pre-climate-change world, Roth explains. “But as the climate stressors change and that infrastructure is subjected to greater heat than was anticipated or larger changes in hydrological conditions, it may be resilient to all sorts of external loadings — but not resilient to changes in climate stressors.”
One expert says that given the unpredictable nature of climate change, it might be best to design infrastructure for a shorter design life, rather than a longer one, with the expectation that future civil engineers would then adapt that infrastructure to the changes they are experiencing. Miguel Medina, Ph.D., F.ASCE, professor emeritus of civil and environmental engineering at Duke University, says, “They’re going to have to design structures for temporary conditions and then adjust it in a few years, according to the new conditions.”
Changing the codes
One obstacle civil engineers face in trying to design infrastructure that will remain operational in the face of the impacts of climate change is the fact that most codes and standards do not take climate change into account. Ayyub says that matters because many engineers think, “I will design according to codes and standards, and then I’m done!”
Consequently, Ayyub says, “improving codes and standards is ... the most important single activity that will have an impact across the board not only in the United States but also overseas because many countries follow what we do.”
If considerations of the impacts of climate change can be added to codes and standards, “engineers will be obliged to use them in their decisions,” points out Michele Barbato, Ph.D., P.E., CEng, F.SEI, F.ASCE, a professor of structural engineering in the civil and environmental engineering department at the University of California, Davis. Barbato is also co-director of the university’s Climate Adaptation Research Center.
To that end, ASCE, the University of Maryland, and the climate program office at the National Oceanic and Atmospheric Administration recently launched a partnership to “accelerate the move toward more climate-resilient infrastructure for the nation and globally,” explained NOAA administrator Rick Spinrad, Ph.D., in a Nov. 3, 2021, press release. Specifically, the collaboration will work to include NOAA-produced climate data in the development and updating of ASCE codes and standards, which influence the vast majority of building codes in the United States and abroad.
“Our goal is to bring climate information into the nation’s standard-setting process to increase the pace of climate adaptation and reduce design, construction, and maintenance costs as well as the costs of climate-related natural disasters,” Spinrad says.
Ayyub will work to facilitate the partnership, along with colleague Dan Walker, Ph.D., A.M.ASCE, the associate director of the University of Maryland’s Center for Technology and Systems Management and a member of ASCE’s Committee on Adaptation to a Changing Climate. Although the effort is just getting started, Walker notes that the issue has global interest. “People involved in European codes see the same problems,” Walker says. “The challenge is: What will be the statistical mechanism that allows you to understand future conditions that you can use for design?”
Climate change should also be taken into account in ASCE’s Report Card for America’s Infrastructure when determining the grades assigned to individual infrastructure categories, notes Jason Giovannettone, Ph.D., P.E., M.ASCE, a former senior engineer/meteorologist at Dewberry and now the director of climate and sustainability for the charitable organization Sisters of Mercy of the Americas, in Silver Spring, Maryland.
The report card is issued roughly every four years, most recently in 2021. But its grades are based on that no-longer-accurate idea of climate stationarity, adds Barbato, who serves with Giovannettone on the Committee on Adaptation to a Changing Climate. Because the 2021 report card assigned a cumulative grade of C- to 17 infrastructure categories, it means “we’re at most mediocre under the present conditions,” which are changing in ways that are difficult to predict, says Barbato.
Declaring interdependence
The interdependence of infrastructure systems is another idea — critical for resilience — that needs to be incorporated into the report card and other civil engineering resources, says Giovannettone. For example, the transportation sector might seem fairly resilient on its own, but in fact, much of the transportation sector is heavily dependent on the energy sector. Much of the mass transit system requires electricity and, increasingly, so do buses and cars.
“So if the energy sector fails, the transportation sector fails,” explains Giovannettone, who recently co-authored a book that discusses the “ever-increasing interdependence between sectors.” That book, Impacts of Future Weather and Climate Extremes on United States Infrastructure: Assessing and Prioritizing Adaptation Actions, is being published by ASCE. An electronic version is available online, and a print version will be published soon.
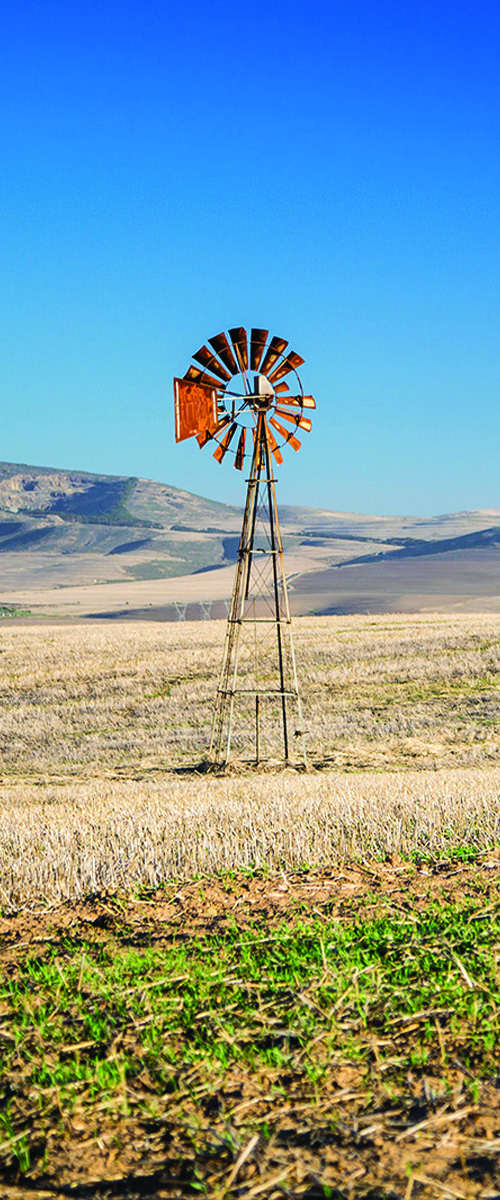
“We wanted to home in on” the topic of infrastructure interdependence because it “isn’t really talked about in many other places,” says Giovannettone. The book project was led by Mari Tye, Ph.D., CEng, MICE, M.ASCE, a project scientist at the National Center for Atmospheric Research in Boulder, Colorado, and the chair of the Committee on Adaptation to a Changing Climate. More than a dozen contributing authors also provided material for the book.
Systems thinking is especially important because some actions intended to bolster infrastructure operations might actually hamper a system’s resilience. For example, when sensors or technology for connected vehicles are installed on highways, the roads become more dependent on their availability and the electricity that powers them, notes Savina Carluccio, CEng, MICE, an associate director in the London office of Arup.
“If you’re overlaying new technology onto existing infrastructure, you might introduce unintended vulnerabilities or dependencies,” says Carluccio, who is also the head of guidance and standards for the Resilience Shift and a program director for the International Coalition of Sustainable Infrastructure, which ASCE helped create.
“You have to understand what impact (that technology) will have on the existing and legacy infrastructure,” Carluccio explains. “So when an adverse event hits and you think the transportation infrastructure is fine, you’ve actually made it dependent on another infrastructure network — you’ve made the system more vulnerable to climate change or other shocks and stresses.”
Changing approaches
What can civil engineers do to prepare themselves for the challenges of designing infrastructure systems that are more resilient to the impacts of climate change?
“One of my favorite quotes about resilience is that ‘You need to prepare to be surprised,’” notes Carluccio. “Uncertainty is one of those things inherent to climate change,” and no one can be prepared for everything. But it is possible to learn from an event, minimize the adverse consequences, and prepare better for the next event — such as by increasing the robustness of infrastructure, developing strategies for a quicker recovery, or identifying means of coordinating actions with customers, stakeholders, or other authorities.
Civil engineers are actually in an “enviable position” and facing the “opportunity of a lifetime,” says Wallace. They have an opportunity to help rewrite the rules as exemplified in the codes and standards. Wallace says, “Engineers can reduce infrastructure project risk, applying their unique knowledge and experience to determine what solutions could work and what won’t work.”
By nature — and often with good reason — “engineers can be risk-averse,” says Field. “So they need to embrace the change that’s coming and try to equip themselves with the new skill sets they’ll need.” These new skill sets are intended to expand the role of civil engineers from strictly technical experts to advisers and consultants to their clients, Field explains. They include communications, critical thinking, and especially a focus on systems thinking.
“The tools of risk analysis will become much more important to civil engineers because we’ll have to deal with deep uncertainty,” notes Roth.
And the ability to conduct climate stress tests will be critical “for a range of climate scenarios to understand what projections actually mean in terms of what happens to a network if certain events hit,” says Carluccio.
Civil engineers can acquire these new skills in various ways — through on-the-job learning or mentoring, internal training programs offered by their employers, online courses provided by ASCE and other engineering organizations, and returning to school to earn a graduate-level degree, Field notes. “The key thing is to keep learning,” she says. “Think about your own personal resilience — how you learn and change and adapt and be willing to do that.”
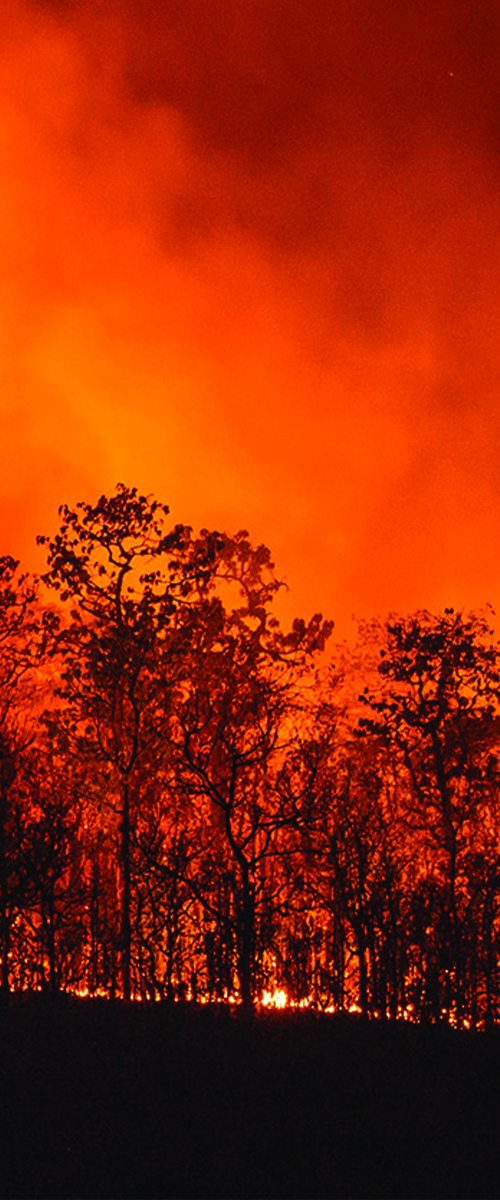
Civil engineers will also need to learn how to work with experts in other fields because the issue of infrastructure resilience to climate change is “not a single-discipline problem … so if you’re only working with engineers, you’re not going to solve it,” notes John W. van de Lindt, Ph.D., F.SEI, F.ASCE, the Harold H. Short Endowed Chair at Colorado State University and the co-director of the Center of Excellence for Risk-Based Community Resilience Planning for the National Institute of Standards and Technology.
Civil engineers must collaborate with mechanical engineers and engineers in other disciplines, architects, urban planners, economists, first responders and other emergency experts, the insurance and reinsurance industries, and experts in the field of social equity. (Read “Can equitable infrastructure investment combat climate change?”)
“The role of the civil engineer will be central in all this,” notes Ayyub. But “when we deal with adaptation and mitigation for a changing climate, it’s certainly a systems problem — it cannot be handled by one specialty or one domain, so there is a need for a collaborative effort.”
Civil engineers need to be involved in the planning stages of projects earlier than they generally are now — admittedly, a long-sought goal of the profession but one that takes on a greater urgency because of climate change. For Roth, civil engineers will “have a new role and a new responsibility to advise owners and regulators” when, for instance, the proposed site of a project is potentially at risk because of climate change.
Civil engineers “can explain their reasons or at least raise awareness that (relocating the project) might offer a significant advantage,” Roth explains. If relocation is not possible, civil engineers can discuss ways to better protect or adapt the site.
And if the health and safety of the public are at risk, civil engineers must also be prepared to walk away from some projects, Roth adds.
Adding things up
Although the idea of resilience to climate change can appeal to clients, the question of how much it will cost arises frequently. “It’s at the heart of climate adaptation,” notes Walker. “If we have additional costs associated with construction, will we see a benefit that offsets that cost?”
Field notes: “Being able to quantify resilience is incredibly important.” Civil engineers must be able to explain “that it will cost you more money in the long run if you don’t do it,” she says.
If infrastructure systems are not adapting to a changing climate and no mitigation is going on, “there are multiple cascading effects and impacts on the economy,” says Wallace. For example, in the Fourth National Climate Assessment — produced for the federal government in 2018 by the U.S. Global Change Research Program — a business-as-usual GHG emissions scenario was compared with a scenario of modest reduction. “Just looking at the cumulated cost of sea level rise and storm surges under the higher (business-as-usual) scenario” was estimated at $3.6 trillion through 2100, Wallace points out. But the scenario in which resiliency measures are implemented would cost just $820 billion, he explains.
Considerable research indicates that every dollar spent on resiliency will generate a return on investment of $4 to $11 “by saving on having to rebuild again and again and again,” says Tye. This is equally true for major events and the so-called nuisance flooding events, which are more frequent than monster storms but can have a cumulative effect that financially exceeds the more extreme events like hurricanes Sandy or Ida, Tye explains.
Although the investments in resiliency can be considerable, the results can be even more dramatic. For example, in the aftermath of Hurricane Katrina in 2005, the city of New Orleans and the federal government spent more than $14 billion designing and constructing new levees and other flood-prevention systems for the Crescent City. But since that system was designed to prevent another Katrina-like scenario — which caused an estimated $160 billion in damages — “I’d say that’s a good investment,” notes Melissa Darr, P.E., M.ASCE, a project water engineer in the Phoenix office of Arcadis, where she also is a member of the firm’s global sustainability team. By all accounts, the new flood defenses in New Orleans did protect the city from the worst of Hurricane Ida in summer 2021.
More recently, the bipartisan $1.2 trillion infrastructure package that was adopted by Congress and signed by President Joe Biden in November 2021 pledged $47 billion for infrastructure resilience, at least some of which is specifically aimed at combating climate change.
“Investing in resilience is almost always going to pay off,” Darr says. But it can be “a tough thing to convince people to do because when you invest in resiliency, the payoff is that nothing happens, and when nothing happens, people aren’t good at remembering that it was an investment we needed to make. What we have to do is convince people to make the investment proactively — and not wait until after the storm.”
Robert L. Reid is the senior editor and features manager of Civil Engineering.
This article first appeared in the January/February 2022 issue of Civil Engineering as “Responding to Code Red.”